A new clock shows how gravity warps time — even over tiny distances
The world’s most precise clock probes space and gravity, too
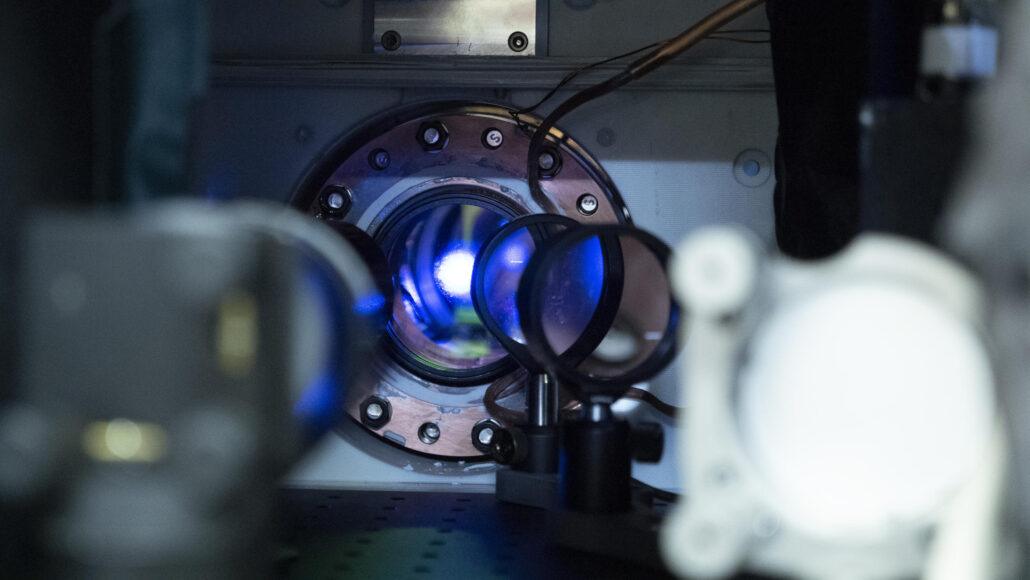
JILA researchers measured time dilation — or how an atomic clock's ticking rate varies with height — within this tiny cloud of strontium atoms (center, white ball).
R. Jacobson/NIST
The force of gravity treats time like taffy. The stronger its pull, the more gravity can stretch out time, making it pass more slowly. By using a new atomic clock, scientists have now measured this slowing of time over the shortest distance yet — just one millimeter (0.04 inch).
Albert Einstein’s theory of general relativity predicts that where gravity is stronger, time passes more slowly. That’s called time dilation. Gravity is stronger closer to the center of the Earth. So, according to Einstein, time should pass more slowly closer to the ground. (And experiments have confirmed this.)
Jun Ye led the research group that now shows how this holds over even super short distances. He’s a physicist at JILA in Boulder, Colo. (That institute was once known as the Joint Institute for Laboratory Astrophysics.) It’s run by the University of Colorado and the National Institute of Standards and Technology.
The new clock’s ability to sense tiny changes in gravity makes it a powerful tool. It could help monitor climate change. It also could help predict volcanic eruptions — even map the Earth. And its design paves the way for atomic clocks that are even more super-precise, its creators say. Such clocks could help solve fundamental mysteries of the universe.
Ye and his colleagues described their findings February 22 in Nature.
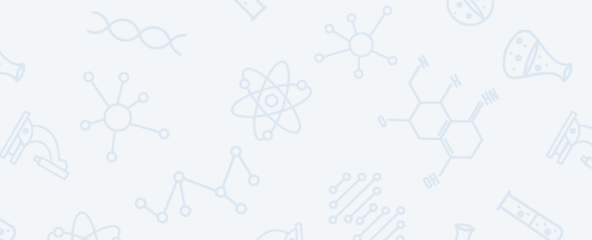
Educators and Parents, Sign Up for The Cheat Sheet
Weekly updates to help you use Science News Explores in the learning environment
Thank you for signing up!
There was a problem signing you up.
Not your grandfather’s clock
The new atomic clock is “a big, dispersed system with a lot of different components,” says Alexander Aeppli. He’s a graduate student on Ye’s team at the University of Colorado. In all, the new clock spans two rooms and contains mirrors, vacuum chambers and eight lasers.
All clocks have three main parts. The first is something that goes back and forth, or oscillates. Then, there’s a counter that tracks the number of oscillations. (That ever-increasing count advances the time shown on the clock.) Finally, there’s a reference against which the clock’s timekeeping can be compared. That reference provides a way to check if the clock is running too fast or too slow.
A grandfather clock is a helpful way to picture how all these parts work together, Aeppli says. It has a pendulum that swings back and forth, or oscillates, at a regular interval — once a second. After each oscillation, a counter moves the clock’s second hand forward. After sixty oscillations, the counter moves the minute hand forward. And so on. Historically, the sun’s position at noon served as a reference to ensure these clocks ran on time.
“An atomic clock has those same three components, but they’re far different in scale,” Aeppli explains. Its oscillations are provided by a laser. That laser has an electric field that cycles back and forth incredibly fast ― in this case, 429 trillion times a second. That’s too fast for electronics to count. So, atomic clocks use a special laser-based device called a frequency comb as a counter.
Because an atomic clock’s fast-ticking laser divides time into such tiny intervals, it can track the passage of time extremely precisely. Such a precise timekeeper requires a super precise reference. And in the new atomic clock, that reference is the behavior of atoms.
At the clock’s heart is a cloud of 100,000 strontium atoms. They’re stacked vertically and held in place by another laser. That laser effectively chills the strontium atoms into optical molasses — a cloud of atoms that are almost completely frozen in place. The clock’s main laser (the one that oscillates 429 trillion times per second) shines on this cloud. When the main laser ticks at the right frequency, the atoms absorb some of its light. Explains Aeppli, that’s how scientists know the laser is cycling at just the right rate — not too fast, not too slow.
Testing Einstein’s prediction
Because the new atomic clock is so precise, it is a powerful tool for measuring gravity’s effect on time. Space, time and gravity are closely related, Aeppli notes. Einstein’s theory of general relativity explained why this should be true.
To test Einstein’s prediction over the smallest height difference yet, the JILA team split the new clock’s stack of atoms in two. The top and bottom stacks were separated by one millimeter. That allowed the scientists to see how fast the clock’s main laser ticked at two different — but very close — heights. This, in turn, revealed how fast time passed at both places.
The researchers found a hundred-quadrillionth of a second difference in time over that distance. At the height of the lower stack, time ran ever so slightly slower than one millimeter above. And that’s just what Einstein’s theory would predict.
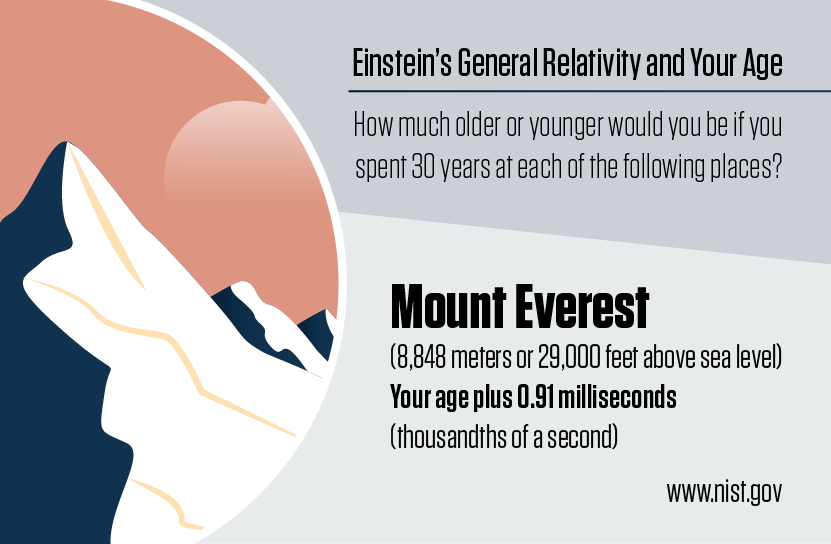
In the past, such measurements required two identical clocks at different heights. For instance, in 2010, NIST scientists used that technique to measure time dilation over 33 centimeters (about 1 foot). The new clock offers a more precise yardstick, Aeppli says. That’s because the height difference between two stacks of atoms in a single clock can be very small and still well-known. “If one were to build two clocks to measure time at different heights, it would be very difficult to determine the vertical distance between the clocks to better than one millimeter,” Aeppli explains.
With the single-clock design, scientists can take images of the upper and lower stacks of atoms to confirm the distance between them. And current imaging techniques, Aeppli notes, allow for separations much smaller than a millimeter. So future clocks could measure the effects of time dilation over even smaller distances. Maybe even as small as the gap between neighboring atoms.
Climate change, volcanoes and mysteries of the universe
“This is truly interesting,” says Celia Escamilla-Rivera. She studies cosmology at the National Autonomous University of Mexico in Mexico City. Such precise atomic clocks can probe time, gravity and space at truly teensy scales. And that helps us better understand the physical principles that govern the universe, she says.
Einstein’s theory of general relativity describes those principles in terms of gravity. That works pretty well — until you get to the scale of atoms. There, quantum physics rules. And that’s a wildly different type of physics than relativity. So, how exactly does gravity fit in with the quantum world? No one knows. But a clock even 10 times more precise than the one used for the new time-dilation measurement could offer a glimpse. And this latest clock design paves the way for that, says Escamilla-Rivera.
Such precise atomic clocks have other potential uses, too. Imagine building a set of reliable and user-friendly atomic clocks, Aeppli says. “You could put them at all the places where you’re concerned about volcanoes erupting.” Before an eruption, the ground often swells or quakes. This would change the height of an atomic clock in the area, and therefore how fast it runs. So scientists might use atomic clocks to detect tiny changes in elevation that signal a possible eruption.
Similar techniques could be used to monitor melting glaciers, Aeppli says. Or, they could improve the accuracy of GPS systems to better map elevations across Earth’s surface.
Scientists at NIST and other labs are already working on portable atomic clocks for such uses, Aeppli says. Those must be smaller and more durable than the ones in use today. The most precise clocks will always be in a lab with well-controlled conditions, he notes. But as those lab-based devices get better, clocks for other applications will, too. “The better we measure time,” Aeppli says, “the better we can do so many other things.”