Particles that zip through matter snare Nobel
Two physicists share the award for demonstrating that neutrinos have mass
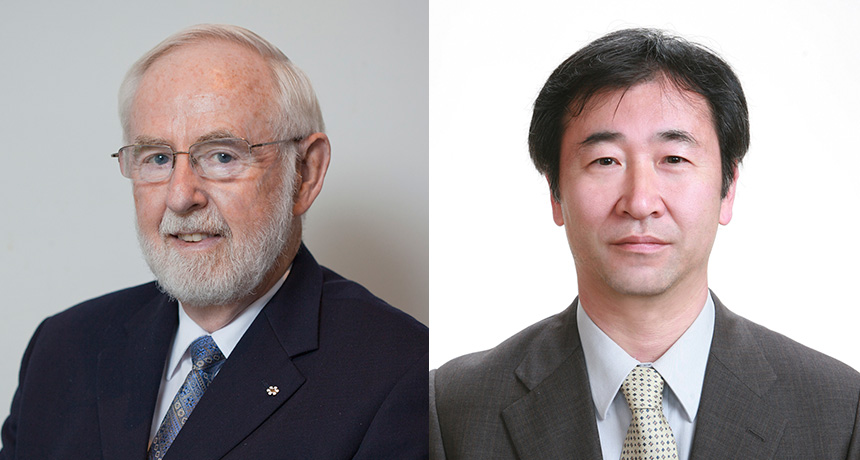
Arthur McDonald of Queen’s University in Kingston, Canada (left), and Takaaki Kajita of the University of Tokyo (right) shared the 2015 Nobel Prize in physics.
FROM LEFT: K. MACFARLANE/QUEEN'S UNIV./SNOLAB; COURTESY OF THE UNIV. OF TOKYO.
By Janet Raloff and Andrew Grant
Every moment, you are being bombarded by particles that can pass invisibly through almost any matter. They even move through you. But no worries: They cause no harm. Called neutrinos, the particles are smaller than atoms. And they are so light that scientists long believed they carry no mass at all. For finding that neutrinos do have mass, two physicists won the 2015 Nobel Prize in physics on October 6. Their discovery is reshaping scientists’ understanding of how the universe works.
Takaaki Kajita of the University of Tokyo in Japan and Arthur McDonald of Queen’s University in Kingston, Canada, shared the award. The scientists led giant underground experiments to detect a few of the neutrinos that pass through Earth. Their experiments showed that the elusive particles switch from one variety into another as they travel. This could only happen if neutrinos have mass. The work confirmed what many physicists had suspected. But it also defies the set of theories that predicts the properties of nature’s particles and forces. Those theories are known as the standard model.
The Nobel news is “incredibly exciting,” says Janet Conrad. She is a neutrino physicist at the Massachusetts Institute for Technology in Cambridge. “I had been waiting for this for so many years.” Neutrino mass is minuscule for individual particles. But it could have major implications for improving the standard model and understanding the evolution of the universe.
The neutrino has been a mystery since its existence was first proposed in 1930.
These particles have been around since the birth of the universe. But they hardly ever bump into other matter. That makes them invisible to most methods of detecting matter. In the 20th century, physicists concluded that neutrinos are massless. They also concluded the particles come in three types, or “flavors.” They named the flavors for the type of particle the neutrinos make when they collide with matter. These collisions can produce electrons, muons and taus. Thus, those are the names of the three flavors.
But there was a problem. The neutrinos weren’t adding up. The sun shoots out torrents of electron neutrinos. But experiments detected only about a third as many as had been expected. Some researchers began to suspect that neutrinos from the sun were oscillating, or switching flavors, on their way to Earth.
Detecting those neutrinos took cleverness and an immense detector. That’s where Kajita and his Super-Kamiokande detector in Japan came in. The underground experiment was switched on in 1996. It consists of more than 11,000 light sensors. The sensors detect flashes of light that occur whenever neutrinos (coming from the sun or anywhere else in the universe) collide with other particles. The collisions all took place inside a tank filled with 50 million kilograms (50,000 metric tons) of water.
Kajita and his coworkers focused on detecting muon neutrinos. These neutrinos are produced when charged particles coming from space collide with air molecules in the Earth’s atmosphere. The researchers counted up the rare flashes from neutrino collisions. Then they traced the neutrinos’ path backward. Their goal was to learn where each one came from.
More muon neutrinos came from above than below, they found. But neutrinos pass through the Earth. That means there should be an equal number coming from all directions. In 1998, the team concluded that some of the neutrinos from below had changed flavors during their trek through Earth’s interior. Like a criminal changing disguises, the muon neutrinos were able to pose as something else — another flavor of neutrino. Those other flavors couldn’t be detected by the muon detector. This behavior, the scientists realized, meant that neutrinos have mass.
In the weird world of neutrino physics, particles also behave like waves. The mass of a particle determines its wavelength. If neutrinos had zero mass, then each particle would act like a single simple wave as it moved through space. But if the flavors have different masses, then each neutrino is like a mix of multiple waves. And the waves are constantly messing with each other and causing the neutrino to switch identities.
The Japanese team’s experiment produced strong evidence for neutrino oscillation. But it couldn’t prove that the total number of neutrinos was consistent. Within a few years, the Sudbury Neutrino Observatory in Canada took care of that issue. McDonald led the research there. His team looked more deeply at the problem of the missing electron neutrinos coming from the sun. They measured the total number of neutrinos coming in. They also looked at the number of electron neutrinos.
In 2001 and 2002, the team confirmed that electron neutrinos from the sun were few and far between. But they showed that the shortage disappeared if neutrinos of all flavors were considered. “There certainly was a eureka moment in this experiment,” McDonald said in a news conference. “We were able to see that neutrinos appeared to change from one type to the other while traveling from the sun to Earth.”
The Sudbury findings solved the missing solar neutrino problem. They also confirmed Super-Kamiokande’s conclusion that neutrinos change flavors and have mass.
The discoveries sparked what Conrad calls the “neutrino oscillation industry.” Experiments probing neutrinos are delivering precise measurements of their identity-changing behavior. These results should help physicists learn the exact masses of the three neutrino flavors. Those masses must be extremely small — about a millionth the mass of an electron. But while tiny, the changeable neutrinos Kajita and McDonald discovered are mighty. And they have had a heavy impact on physics.
Power Words
(for more about Power Words, click here)
atmosphere The envelope of gases surrounding Earth or another planet.
atom The basic unit of an element. Atoms have a nucleus of protons and neutrons, and electrons circle the nucleus.
electron A negatively charged particle, usually found orbiting the outer regions of an atom; also, the carrier of electricity within solids.
flavor (in physics) One of the three varieties of subatomic particles called neutrinos. The three flavors are called muon neutrinos, electron neutrinos and tau neutrinos. A neutrino can change from one flavor to another over time.
mass A number that shows how much an object resists speeding up and slowing down — basically a measure of how much matter that object is made from. For objects on Earth, we know the mass as “weight.”
matter Something which occupies space and has mass. Anything with matter will weigh something on Earth.
molecule An electrically neutral group of atoms that represents the smallest possible amount of a chemical compound. Molecules can be made of single types of atoms or of different types. For example, the oxygen in the air is made of two oxygen atoms (O2), but water is made of two hydrogen atoms and one oxygen atom (H2O).
neutrino A subatomic particle with a mass close to zero. Neutrinos rarely react with normal matter. Three kinds of neutrinos are known.
oscillate To swing back and forth with a steady, uninterrupted rhythm.
radiation One of the three major ways that energy is transferred. (The other two are conduction and convection.) In radiation, electromagnetic waves carry energy from one place to another. Unlike conduction and convection, which need material to help transfer the energy, radiation can transfer energy across empty space.
standard model (in physics) An explanation of how the basic building blocks of matter interact, governed by the four fundamental forces: the weak force, the electromagnetic force, the strong interaction and gravity.
subatomic Anything smaller than an atom, which is the smallest bit of matter that has all the properties of whatever chemical element it is (like hydrogen, iron or calcium).
theory (in science) A description of some aspect of the natural world based on extensive observations, tests and reason. A theory also can be a way of organizing a broad body of knowledge that applies in a broad range of circumstances to explain what will happen. Unlike the common definition of theory, a theory in science is not just a hunch. Ideas or conclusions that are based on a theory — and not yet on firm data or observations — are referred to as theoretical. Scientists who use mathematics and/or existing data to project what might happen in new situations are known as theorists.