The Mentos geyser: From demo to real science
I moved a popular party trick to the laboratory to make it more informative
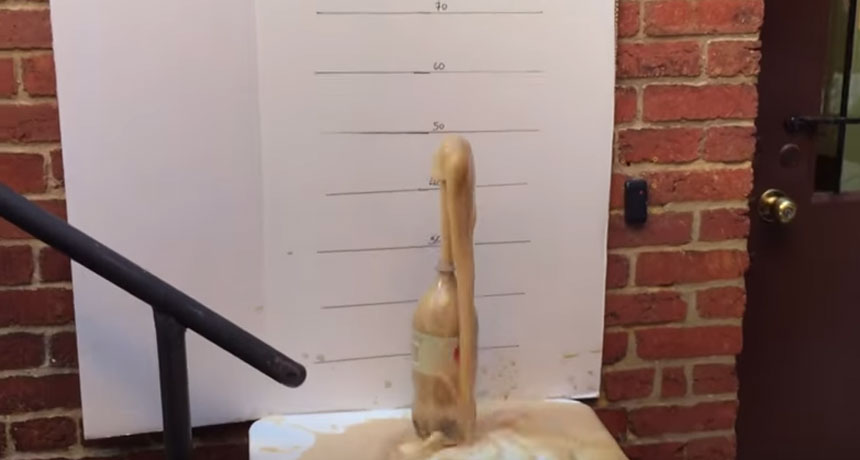
Soda geysers are an explosive good time, but you need to vary your sweets if you’re going to turn a demonstration into an experiment.
B. Bello/SSP
This article is one of a series of Experiments meant to teach students about how science is done, from generating a hypothesis to designing an experiment to analyzing the results with statistics. You can repeat the steps here and compare your results — or use this as inspiration to design your own experiment.
If you’re ever up for a fun and messy time, take one two-liter bottle of diet soda outside. Open the cap and place it on the ground. Use a small paper tube to quickly slide in 6 Mentos mint candies. Then, make way!
The resulting geyser is one of the most popular science demonstrations. But it’s not an experiment. Despite its explosive power, it doesn’t answer questions about how the geyser works or what aspects of its ingredients matter most. Luckily, it’s easy to turn this demonstration into your own experiment. I’ll show you how.
What gives the soda geyser its energetic gush? When people talk about this demonstration on the Internet, they always use Mentos. They are very specific about the candy — it has to be Mentos.
I looked up other science sites to find out why Mentos are important. By reading some of the scientific papers on this, I learned that Mentos are covered in tiny little pits. This allows bubbles to form, triggering our fountain of fizz.
Behind those bubbles, I learned, is a process called nucleation. In chemistry, this process causes structures to form by themselves. In the case of the soda spray, it’s the bubbles of carbon dioxide that assemble by themselves. Using high pressure, beverage makers pump this colorless, odorless gas into a drink to provide a pleasant tingle. That gas remains dissolved in the liquid until the soda container is opened. This releases the pressure. At once, carbon-dioxide molecules begin to collect as tiny bubbles.
Usually this bubble formation — nucleation — isn’t very explosive. But that changes when you add a little bit of candy. Mentos have a surface full of tiny craters. The little bumps and dips serve as nucleation sites— spots where tiny carbon-dioxide bubbles can cling. With all those craters, bubbles form so fast they need to explode outward. And in a bottled up soft drink, they have nowhere to go but up.
Knowing how the geyser works gives me a way to form a hypothesis — an idea I can test. If nucleation sites are important to form a geyser, then I might hypothesize that candies with rough surfaces will produce bigger fountains than candies with smooth surfaces.
I decided to test three kinds of sweets. As my control, I’ll again use those Mentos, with their pitted surfaces. Next, I’ll try M&Ms, which have a very smooth candy shell. Finally sugar cubes have pits and craters much larger than the Mentos.
These three sweets also start out as different sizes. So instead of putting in the same numbers of candies, I will put in the same mass — 22 grams (about 0.8 ounces) of each type.
I measured fountain size in two ways. First, I set off all my geysers in front of a wall. I put marks up the wall every 10 centimeters (or 3.9 inches). I then videotaped all of my geysers to identify their maximum height.
But there’s another way to measure geysering: weigh the soda! A soft drink that erupts with a high spout will lose more of its liquid than one that emits a small squirt. By weighing the soda bottles before and after each test, I can determine how much liquid was lost in each test.
I also took care to test each type of candy many times. Each fountain spray will be slightly different, even if each test uses the same kind and amount of candy. For each type of candy — my test variable — I added up all of the geyser sizes and calculated the average. Then I ran statistics, comparing the average geyser size for each type of candy.
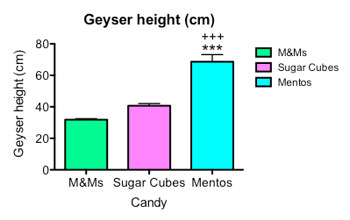
I made six fizzing fountains for each type of candy. Then I graphed my results, so that everyone can see my data.
You can see on the right that M&Ms, with the smoothest shell, triggered the smallest eruption, measured by loss of beverage mass. Sugar cubes made a bigger one. Mentos made the biggest of all. The stars and plus signs on my graph are used to indicate a p value —the probability of seeing a difference as big as the one I found if the difference between geysers was due to chance. These stars and plus signs indicate a very small p value of less than 0.001 — or less than one percent — suggesting the result was significant, and not due to chance.
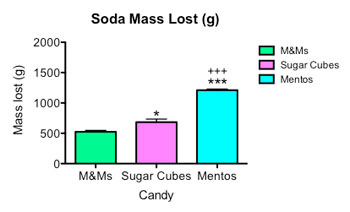
I also weighed my sodas before and after. In the second graph at right you can see that the sodas with M&Ms lost less weight than sodas with sugar cubes. Mentos geysers spouted out the most soda.
Finally I performed a correlation — or comparison identifying a possible link — between geyser height and the amount of soda lost during a geyser.
The graph below shows there is a relationship between the height of the geyser and the amount of weight lost from each soda bottle during the test. A taller geyser is associated with a larger loss in soda mass — a positive correlation.
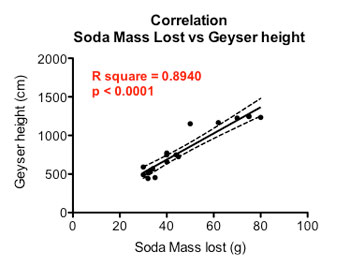
Have you ever done a soda geyser? Turn a simple fountain into an experiment, and see if you can repeat my results.
Note: The idea for this experiment came from the great book Candy Experiments 2, by Loralee Leavitt. This book has loads of chemistry demonstrations, all featuring candy!
Follow Eureka! Lab on Twitter
Power Words
(for more about Power Words, click here)
carbonation The process of pumping carbon dioxide into a liquid, to imbue that liquid with bubbles. It gives the now-carbonated liquid a fizzy taste.
carbon dioxide A colorless, odorless gas produced by all animals when the oxygen they inhale reacts with the carbon-rich foods that they’ve eaten. Carbon dioxide also is released when organic matter (including fossil fuels like oil or gas) is burned. Carbon dioxide acts as a greenhouse gas, trapping heat in Earth’s atmosphere. Plants convert carbon dioxide into oxygen during photosynthesis, the process they use to make their own food.
correlation A mutual relationship or connection between two variables. When there is a positive correlation, an increase in one variable is associated with an increase in the other. (For instance, scientists might correlate an increase in time spent watching TV with an increase in risk of obesity.) Where there is an inverse correlation, an increase in one value is associated with a decrease in the other. (Scientists might correlate an increase in TV watching with a decrease in time spent exercising each week.) A correlation between two variables does not necessarily mean one is causing the other.
geyser A vent (opening) in Earth’s surface that intermittently sends up a tall spray of steam or hot water. The sometimes explosive discharge of water and steam is propelled by the geothermal heating of water below ground.
hypothesis A proposed explanation for a phenomenon. In science, a hypothesis is an idea that must be rigorously tested before it is accepted or rejected.
mass A number that shows how much an object resists speeding up and slowing down — basically a measure of how much matter that object is made from. For objects on Earth, we know the mass as “weight.”
molecule An electrically neutral group of atoms that represents the smallest possible amount of a chemical compound. Molecules can be made of single types of atoms or of different types. For example, the oxygen in the air is made of two oxygen atoms (O2), but water is made of two hydrogen atoms and one oxygen atom (H2O).
nucleation (in chemistry) The process of new structures forming by self-assembly, or the start of a new phase in a chemical process that happens spontaneously.
p value (in research and statistics) This is the probability of seeing a difference as big or bigger than the one observed if there is no effect of the variable being tested. Scientists generally conclude that a p value of less than five percent (written 0.05) is statistically significant, or unlikely to occur due to some factor other than the one tested.
statistical analysis A mathematical process that allows scientists to draw conclusions from a set of data. In research, a result is significant (from a statistical point of view) if the observed difference between two or more conditions is unlikely to be due to chance. Obtaining a result that is statistically significant means that it is unlikely to observe that much of a difference if there really is no effect of the conditions being measured.
statistical significance In research, a result is significant (from a statistical point of view) if the likelihood that an observed difference between two or more conditions would be due to chance. Obtaining a result that is statistically significant means there is a very high likelihood that any difference that is measured was not the result of random accidents.
statistics The practice or science of collecting and analyzing numerical data in large quantities and interpreting their meaning. Much of this work involves reducing errors that might be attributable to random variation. A professional who works in this field is called a statistician.