Astronomers finally find the cosmic source of gold and silver
Gravitational waves point to answers for some longstanding mysteries of the universe
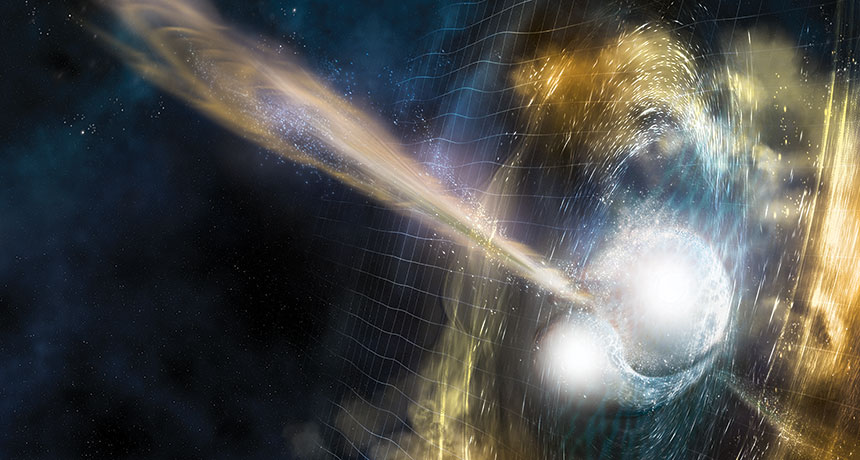
A smashup of two neutron stars is shown in this artist’s rendering. Such a collision sent out gravitational waves and a burst of gamma rays. The ejected debris also left a glow.
NSF, LIGO, A. Simonnet/Sonoma State Univ.
WASHINGTON, D.C. — Some 130 million years ago, the ultradense cores of two dead stars collided. The first evidence of the cataclysmic smashup were gravitational waves. They reached Earth on August 17. As astronomers rushed to home in on their source, they turned up a trove of riches. It is helping explain, among other things, the source of such precious metals as silver, gold and platinum.
This smashup marked the first direct sighting of a collision between two neutron stars. Researchers announced the event today at a news conference in Washington, D.C. Neutron stars are the remnants of aged stars that died in an explosion. The corpses of these stars are spectacularly dense. A single teaspoon of such neutron-rich material would carry a mass that on Earth would weigh roughly one billion tons.
Churning debris produced in the afterglow of the collision included newly created gold, silver and platinum. There was also a smattering of other heavy elements. Among those: uranium. Until now, the birthplace of such elements had been unknown.
“It really is the last missing piece” of the periodic table, says Anna Frebel. An astronomer, she works at the Massachusetts Institute of Technology, in Cambridge. She was not involved in the new research.
Story continues below image.
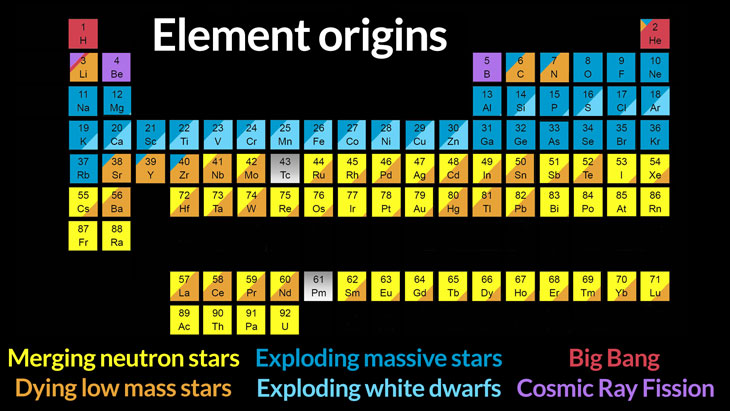
The extreme conditions produced in the newfound collision forged heavier elements than the parent stars had hosted. “This is heaven for anyone working in the field,” Frebel says. And this new-element factory was quite effective. For instance, scientists have just calculated that the smashup produced about 10 to 100 times Earth’s mass in gold!
It took a scientific village
To probe the event, scientists used data gathered from about 70 different observatories. What they learned from poring over those data resulted in at least 20 new research papers. All were released today. Included among their data had been a tremor of gravitational waves. The Advanced Laser Interferometer Gravitational-Wave Observatory, or LIGO, spotted it two months ago. This was the first sign of the smashup.
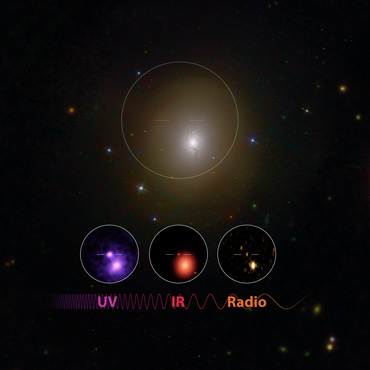
The National Science Foundation funds LIGO. Observes France Córdova, NSF’s director, “Already [LIGO] is transforming our understanding of the universe.” She says it’s offering “a fresh narrative of the physics of stars in their death throes.”
Like musical instruments taking turns in a symphony, a sequence of various types of electromagnetic radiation (light) followed that first gravitational trill. It started with a burst of gamma rays. Then came a glow of visible and infrared light. These were first spotted about 12 hours after the gravity waves. More than a week later, as those wavelengths faded away, X-rays crescendoed. Radio waves then followed.
Astrophysicists have long dreamed of being able to combine gravitational waves with light to study a neutron-star merger. “The picture that you can put together by having all of those sources is synergistic,” says David Shoemaker. By that he means it’s greater than the sum of the parts. Shoemaker, at MIT, is a spokesperson for LIGO.
It started with gravity waves
LIGO consists of two detectors. One sits in Washington State, another in Louisiana. Both registered an unmistakable sign of the upheaval. It was a shimmying of space itself. It lasted for about 100 seconds before cutting off. It was the strongest and longest series of spacetime ripples LIGO had ever seen.
At once, scientists knew they had something big, says Vicky Kalogera. She’s a LIGO member at Northwestern University in Evanston, Ill. Emails quickly circulated, she said, saying “Oh my God, this is it.”
That ripple in spacetime was an indication of a cosmic crash. As if on an ill-fated merry-go-round, two orbiting neutron stars whirled around each other. They spiraled closer and closer. Eventually, they merged. Each neutron star had a mass a bit greater than that of the sun. The pairing probably collapsed into a black hole (although LIGO scientists can’t be certain). LIGO has previously spotted mergers of swirling black holes having masses tens of times that of the sun. The smaller masses of the orbiting duo suggested that this time the smashup involved neutron stars. And because black holes aren’t expected to emit light, the fireworks that followed confirmed neutron stars as the source.
A sister gravity wave detector, Virgo, exists in Italy. It saw only a faint signal. That helped narrow down where to look for signs of that smashup. It was “a part of the sky that was a blind spot of Virgo,” Kalogera says. Knowing that helped astronomers find the event’s general location in the southern sky.
Just two seconds after the gravity-wave signal, NASA’s Fermi space telescope spotted a glimmer of gamma rays in the same part of the sky. Quickly, other telescopes swung into action. One by one, they began picking up a glow where none had been.
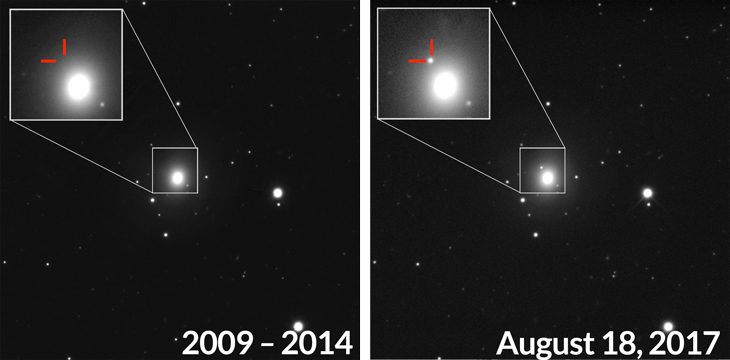
“We saw what looked like a new star,” says Edo Berger. He’s an astronomer at Harvard University in Cambridge, Mass. He led a team in South America that spotted the light using a camera on the Blanco telescope in Chile. His was one of several teams that observed the blast’s light. That detection pinpointed in which galaxy the collision had occurred. It was NGC 4993. The actual smashup now appears to have taken place 130 million light-years from Earth in the constellation Hydra.
“There was this moment of disbelief” after putting all of that data together. Then, Berger recalls thinking, “Wow, we actually did it. We found it!”
Precious element factory
That afterglow also revealed the birth of elements. As the collision spurted neutron-rich material into space, a variety of heavy elements formed through a chain of nuclear reactions known as the “r-process.” It requires an environment crammed with neutrons. When the conditions are right, atomic nuclei rapidly gobble up neutrons and then undergo radioactive decay. This transforms old elements into newer, bigger ones, before they resume their neutron gorge-fest. The r-process is thought to produce about half of all elements heavier than iron.
In follow-up observations, astronomers picked up the characteristic glow of this process. It’s known as a kilonova (KIL-uh-NO-vuh). “Until this event, we had never directly seen anywhere in nature these heavy elements being forged. Now we have,” says Brian Metzger. He’s a theoretical astrophysicist at Columbia University in New York City. Suddenly, he says, it seems “like you’ve discovered some kind of secret of nature.”
Astrophysicists had disagreed about where the r-process takes place. Two top candidates were exploding stars, called supernovas, and neutron-star mergers. Although scientists can’t yet say whether all r-process elements come from colliding neutron stars, the amount of heavy elements that such smashups should produce appears large enough to explain the amounts of these heavy elements that exist in our universe.
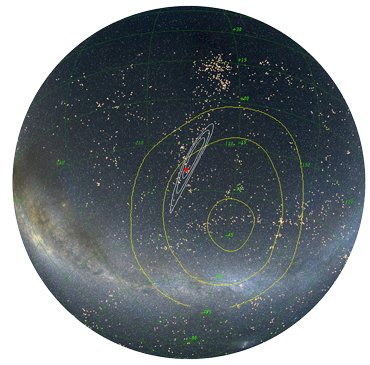
The collision also yielded something known as a short gamma-ray burst. This brief spurt of high-energy light lasted less than two seconds. Such bursts appear in the sky about 50 times a year. Understanding where they come from has been “a long-standing problem in astrophysics,” says Rosalba Perna. She’s a theoretical astrophysicist at Stony Brook University in New York. The new data have now clinched it: Short gamma-ray bursts come from neutron star mergers.
By studying how the neutron stars spiraled inward, astrophysicists also got their first chance to test the “squishiness” of neutron-star material. Scientists don’t fully understand how that material responds when squeezed. Although the results couldn’t pin down whether the neutron stars were squishy, some theories had predicted they could be ultrasquishy. The new data rule that out.
Finally, the new union of neutron stars allowed researchers to gauge the rate at which the universe is expanding. They did it by measuring the distance of the collision using gravity waves. Then they compared this to how much the wavelength of light from the galaxy was stretched by that expansion.
Scientists had measured this property — known as the Hubble constant — before. But they used other means. And those measurements disagreed with each other. That puzzled scientists. Now, scientists have “a totally different, independent measurement,” says Daniel Holz. He’s a LIGO-collaboration member at the University of Chicago in Illinois. The new measurement indicates that distantly separated galaxies are spreading apart at about 70 kilometers (43.5 miles) per second for each megaparsec between them. (A megaparsec is a distance in space equal to 3.3 million light-years.) The new estimate falls squarely between the two previous estimates. And future neutron-star mergers could help improve the measurement.
“These are all just unbelievable, major advances,” Holz says. “It’s really been this insane thrill!”
The excitement has yet to die down. Take it from astronomer Ryan Foley of the University of California, Santa Cruz. His team was the first to spot visible light from the merger. And he says: “This is certainly the biggest discovery of my career and probably will be the biggest discovery of my entire life.”
Helen Thompson/Science News/YouTube