Brains may need flexible networks to learn well
The brain’s ability to shift connections might boost learning
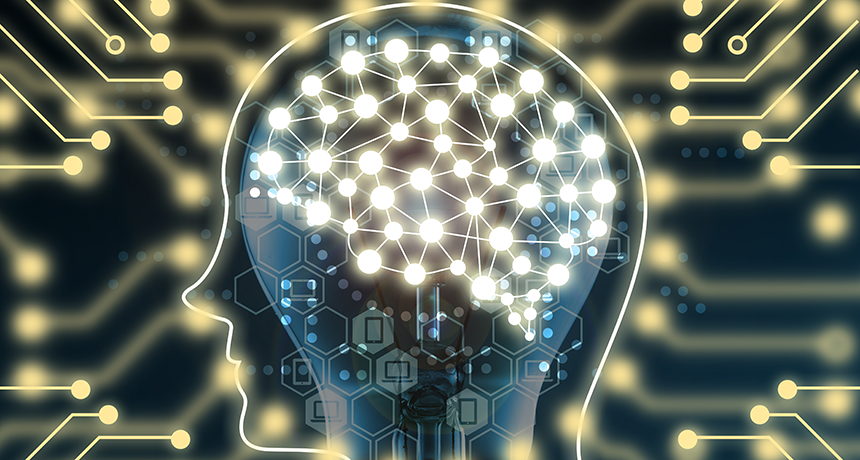
In brains that learn with ease, groups of nerve cells switch easily between conversations with other cells in their networks.
Zapp2Photo/iStockphoto
Look inside the brain of someone learning. You just might be lucky enough to spy a new bridge between two nerve cells pop into existence. Called a synapse, this bridge cements new knowledge into the brain. As new information arrives, some synapses form and strengthen. At the same time, others weaken. But that’s okay. This makes way for more new connections.
You might see more subtle changes, too. These could include changes in the levels of signaling molecules. You might even glimpse slight boosts in the activity of nerve cells, also known as neurons.
Over the last few decades, scientists have zoomed in on these microscopic changes that happen as brains learn. That detailed scrutiny has revealed a lot about the synapses that wire our brains. But it isn’t enough. Brain scientists still lack a complete picture of how brains learn.
Some scientists now think that maybe they have just been looking too closely. When it comes to the science of learning, zeroing in on synapse action risks missing the forest for the trees.
A new, zoomed-out approach attempts to make sense of large-scale brain changes that underlie learning. By studying the shifting interactions between many different brain areas over time, scientists are beginning to grasp how the brain takes in new information — and holds onto it.
Such studies rely on powerful math. Brain scientists are turning to approaches developed in other network-based sciences. They also are borrowing tools that reveal in precise, numerical terms the shape and function of the brain-signaling pathways that shift as people learn.
Danielle Bassett is one of these network neuroscientists. She works at the University of Pennsylvania in Philadelphia. “When you’re learning, it doesn’t just require a change in activity in a single region,” she notes. “It really requires many different regions to be involved.” Her whole-brain approach asks: “What’s actually happening in your brain while you’re learning?” Bassett is charging ahead to both define this new field of “network neuroscience” and =to push its boundaries.
Such work “is very promising,” says Olaf Sporns. He’s a neuroscientist at Indiana University in Bloomington. Bassett’s research, he says, holds the potential to bridge gaps between what brain-imaging studies show and what scientists understand about how learning occurs. “I think she’s very much on the right track.”
Story continues below image.

Already, Bassett and others have found hints that the brains that learn best have flexible networks. By that she means that they are able to rewire connections on the fly. This allows new knowledge in. Some brain regions almost always communicate with the same neural partners. Others show great flexibility. They can quickly swap who they’re talking to. Think of them like people who can send a holiday-party invite to neighbors and friends on their e-mail lists, then moments later shoot off a memo to colleagues at work.
In a few studies, researchers have witnessed this flexibility in action. They put volunteers into brain scanners and then taught them something new. As the scan recorded their brain activity, the scientists could watch networks rewire themselves. Such network flexibility may aid in several types of learning. Too much flexibility, however, could signal certain types of brain disease, other studies suggest.
Not surprisingly, some researchers are rushing to apply this new information. They are testing ways to boost brain flexibility for those of us who may be too rigid in our neural (nerve cell) connections.
“These are pretty new ideas,” says Raphael Gerraty. He’s a cognitive neuroscientist at Columbia University in New York City. The math and computer tools required for this type of research didn’t exist until recently, he notes. So people hadn’t been thinking about learning from such a network-scale perspective. There was simply a technological roadblock, he says. But the road at last is clear and “people can now explore.”
It takes a neural village
That conceptual path is more of a map. It is made of countless neural roads. Even when someone learns something very simple, large swaths of the brain jump in to help. Consider learning an easy sequence of movements, like tapping out a brief tune on a keyboard. This prompts activity in a part of the brain that directs finger movements. The action also calls into play brain areas involved in vision, decision-making, memory and planning. And finger taps are a pretty basic type of learning. In many situations, learning calls up even more brain areas. It gets information from multiple sources to weave together, Gerraty says.
He and his colleagues have seen glimpses of such interactions by scanning the brains of people who had learned to associate two faces. Only one of the faces was paired with a reward. In later experiments, the researchers tested whether people could figure out that the halo of good fortune linked with that one face also extended to the face it had been partnered with earlier. This process is known as “transfer of learning.” And it is something that people do all the time (such as when someone may be wary of the salad at a restaurant that recently served tainted cheese).
Particular brain signatures showed up in study participants who were good at applying knowledge about one thing — in this case, a face — to a separate thing. It’s something that Gerraty and his colleagues reported three years ago in the Journal of Neuroscience. They looked at links between the hippocampus (a brain structure important for memory) and the ventromedial prefrontal cortex (involved in self-control and decision making). Those links were weaker in good learners than in people who struggled to learn. Brain scans performed several days after the learning task revealed differences between those brains, the researchers say. The experiment also turned up differences in the neural networks among these regions and in larger-scale networks that span the whole brain.
Vinod Menon is a neuroscientist at Stanford University in California. In 2015, he scanned the brains of children who have difficulty learning math. And his team turned up unexpected brain connectivity in them. Compared to kids without these problems, the math-challenged kids had more neural connections. This showed up particularly within regions of their brains involved in solving math problems.
That overconnectivity was a surprise, Menon says.
Earlier work had suggested that the math-related networks were too weak to process information well. But the new data suggest an alternative explanation. Too many links may create a system that can’t handle too much new information. In the end, he says, the result may be that “it’s not going to be as responsive.” His team described its findings in Developmental Science.
There needs to be a balance, Menon says. Neural pathways that are too weak can’t carry necessary information. Pathways that are too connected don’t allow new information in. But there’s more to the problem than that. It seems that connections in some areas are more important than in others. And which ones matter seem to depend on the task.
Neural networks need to shuttle information around quickly and smoothly. To really get a sense of this movement — as opposed to snapshots frozen in time — scientists need to watch the brain as it learns. “The next stage is to figure out how the networks actually shift,” Menon says. “That’s where the studies from Dani Bassett and others will be very useful.”
Flexing in real time
In a 2015 study, Bassett and her colleagues described changes to networks as people learned. They gave volunteers simple sequences to tap out on a keyboard. This happened while each person was undergoing a functional MRI scan. This technology can study changes in the brain as people are doing things. The study scanned people on and off over a six week period as they learned the new task. And those scans showed that learning seemed to shift around some of the neural networks in their brains.
Some connections strengthened. Others weakened.
Bassett and her team reported their findings in Nature Neuroscience.
People who learned quickly to tap the correct sequence of keys showed an interesting neural trait. As they learned, they shed certain connections between two areas of their brains. One was the frontal cortex. This is the outermost layer of the brain toward the front of the head. The other area is the cingulate. It sits toward the middle of the brain.
A connection between these brain areas has been linked with the ability to direct attention, set goals and make plans. These are skills that may be important for the early stages of learning but not for later ones, the researchers now suspect. Compared with slow learners, fast learners were more likely to have shed these connections. And this may have made their brains more efficient.
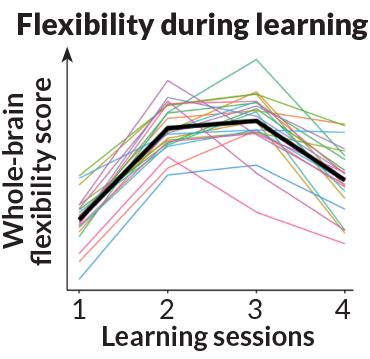
Flexibility seems to be important for other kinds of learning too, Gerraty, Bassett and others reported online May 30 at bioRxiv.org. One example: reinforcement learning. This is where right answers get a thumbs up and wrong answers are called out. This network comprises many points in two areas of the brain. One is the cortex, the brain’s outer layer. The other is a deeper structure known as the striatum (Stry-AY-tum). Last year, Bassett and her colleagues published a paper showing that some brain regions involved in language comprehension also have the ability to quickly form and break connections.
Such studies that watch a brain in action, Gerraty says, show how changeable its networks are during learning. Certain parts disconnect from partners and quickly join new ones. In other words, the act of learning takes flexibility.
But too much flexing may be bad.
Schizophrenia is a disabling mental disorder in which people may feel they lose touch with the outer world. They can suffer from hallucinations or delusions. They may think people are out to get them (when they aren’t). They may have memory problems or trouble paying attention to things. They may not express emotions. They may have trouble making good decisions (ones that are in their best interests). It’s a very serious disease. And while performing a memory task in a scanner, people with schizophrenia show higher flexibility among neural networks across the brain than did healthy people. Bassett and her colleagues reported this, last year, in the Proceedings of the National Academy of Sciences.
Story continues below image.
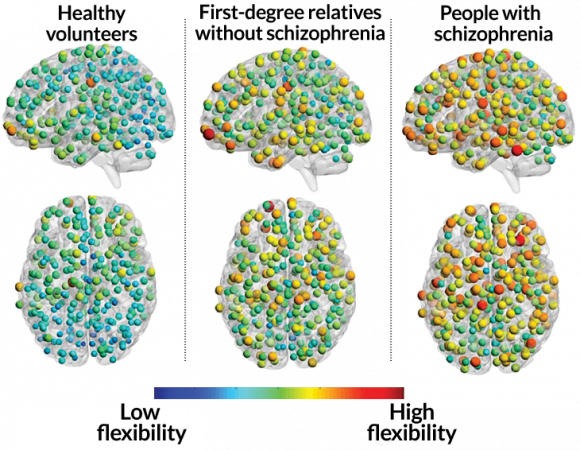
Just how this flexibility arises, and what controls it, is unknown.
Push for more
For now, some researchers are charging ahead, looking for signs that neural flexibility might offer a way to boost one’s ability to learn new things.
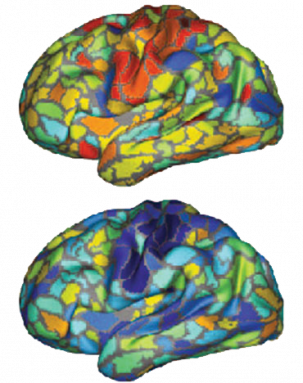
After researchers stimulated a region of the brain known as a “memory circuit,” people were better able to recall lists of words. Scientists described their success with this May 8 in Current Biology. If stimulation can boost memory, some scientists now argue, the way it works may involve enhancing that network flexibility. It might also aid in learning things in the first place.
Certain drugs also show promise. One, known as DXM, is found in some cough medicines. It blocks certain proteins in the brain, helping to control nerve-cell chatter. The compound appears to do this in healthy people by making some of their brain regions more flexible and better able to rapidly switch partners. Bassett’s team reported this last year in the Proceedings of the National Academy of Sciences.
Neural flexibility may also be connected to mood. On March 31 in Scientific Reports, Bassett and her team described analyses of one person: a neuroscientist. He submitted to three brain scans a week for a year. Each time, he described his mood. The standout result: When this man was happiest, his brain was most flexible. The reasons aren’t yet clear. (Flexibility was lowest when he was surprised.)
This research is just getting started. But already, insights on learning are coming quickly from the small group of researchers who are viewing the brain as a matrix of nodes and links — ones that deftly shift, swap and rearrange themselves. The initial findings on network flexibility and learning,” Bassett says, suggest “a whole new set of hypotheses and new ways of testing them.”