Zap, zap, zap! Our bodies are electric
Electric currents race through the brain, keep the heart on pace and strengthen bones
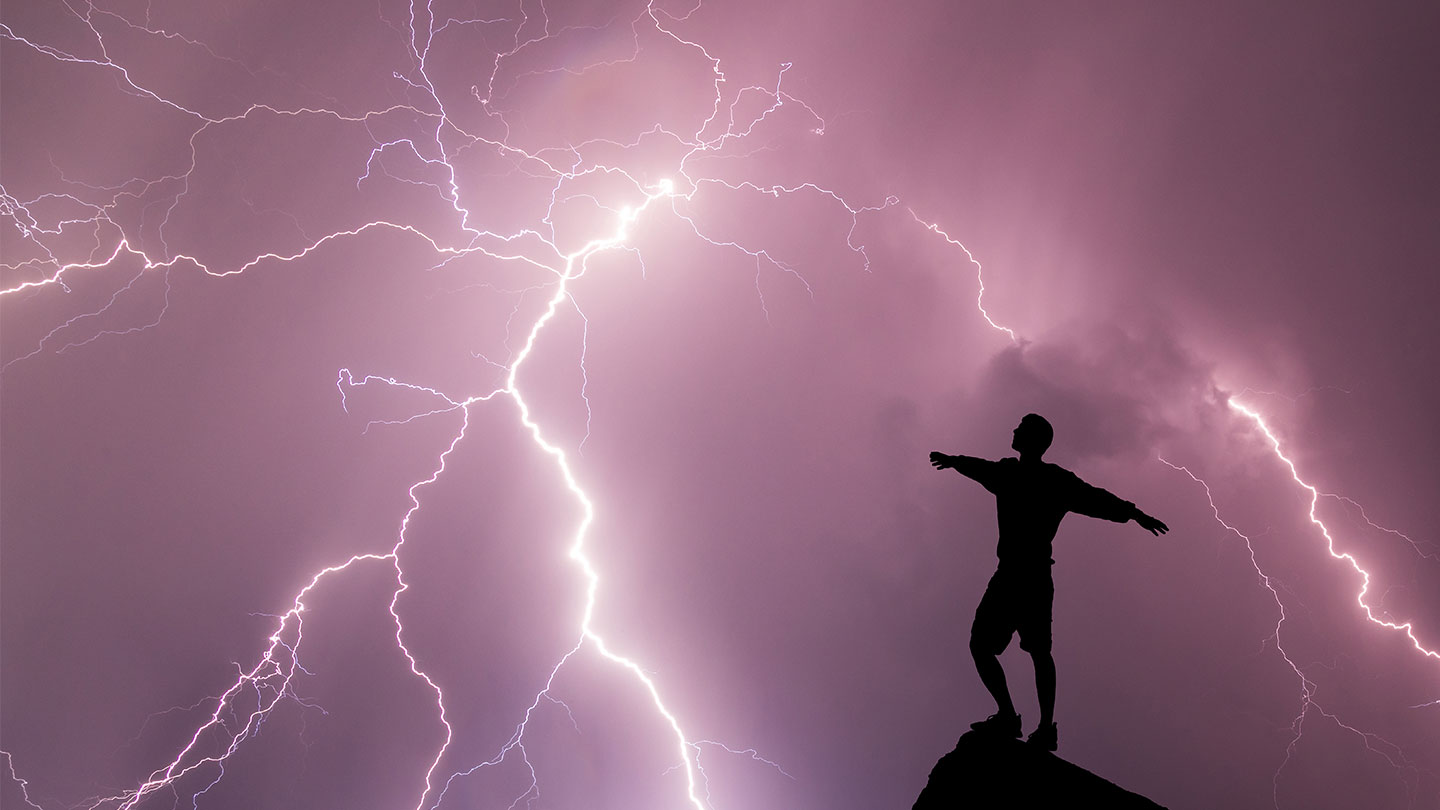
We think of electricity as a big arc of charge, like the dramatic sparks of lightning in a thunderstorm (but don't stand in one!). But far tinier zaps drive processes throughout the body. They literally keep us alive.
Anton Petrus/Moment/Getty Images Plus
Our daily lives seem to run on electricity. Electric lights illuminate our rooms. Electric power runs our computers, microwave ovens, cell phones and countless features on our cars. When we lose access to electricity, it can feel like we also lose access to much of the modern world.
But there’s one place where electricity never stops — your body.
When asleep or resting quietly, your heart beats between 60 and 100 times per minute.
When you’re angry, working out or scared, it beats faster. What helps all the heart’s millions of cells contract rhythmically over and over? And what triggers a change in the pace of that? Electricity.
Many of our cells are tiny generators of electric charge. Working together, they power systems that keep our bodies going. Indeed, every second of every day, electrical signals race through your brain, heart and muscles.
By learning more about these tiny electrical signals, scientists are on the road to ushering in new treatments for when the body doesn’t work quite as well as it should.
Ions charge up cells
The pacing of heatbeats comes from a bundle of cells smaller than a coffee bean. It sits at the top of the heart’s upper right chamber. Called the sino-atrial (or SA) node, this is the heart’s pacemaker.
Natural pumps shove charged atoms — called ions — through the cells’ membranes. Their movement concentrates various types of ions on the inside and outside of each cell.
An ion carries a tiny electrical charge. So concentrating lots of ions on one side of a cell’s membrane will give that membrane an overall electrical charge. There are positive ions on both sides of these membranes. SA node cells pump out positively charged sodium ions. As a result, the outside of their membrane has an overall positive charge.
Cells of the SA node also pump positively charged potassium ions to their insides. But the potassium ions are competing with many other molecules inside the cell, most of which have a negative charge. So at rest, a pacemaker cell is more negatively charged inside than out.
To trigger a heartbeat, pacemaker cells in the SA node open channels at one site in their membranes. Because there are more sodium ions on the outside, those ions will rush inside the cell. They’re trying to balance the electrical charges on either side. That ion flow changes the electric charge across the membrane.
Now other channels in the cell’s membrane open. This allows potassium ions to go racing out. Again, they’re trying to balance their concentrations inside and outside of the cell.
Each channel set opens in one tiny area of a cell’s membrane. Ions that rush into the cell change the electric charge a little ways away. This triggers more channels to open farther down the membrane, sending waves of changing electric charge down the length of a cell.
Scientists refer to this zap as an “action potential.”
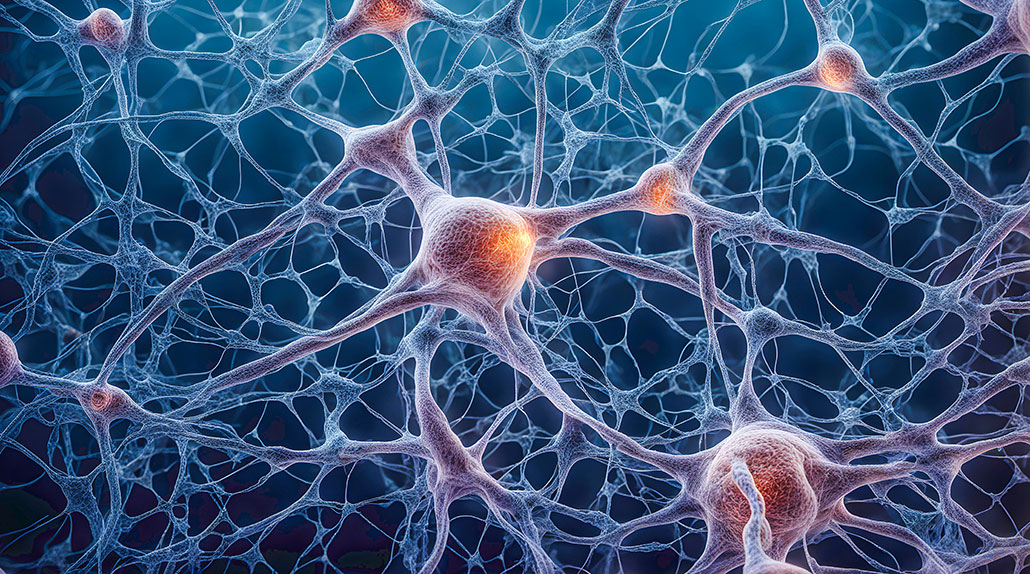
The natural pacemaker’s ‘clocks’
Cells in the SA node all zap at once. Each collective zap is like the beat of a metronome.
Two tiny clocks set the pace at which this metronome beats, explains Edward Lakatta. He’s a cardiologist, or doctor who studies and treats diseases of the heart. He works at the National Institutes of Health Laboratory of Cardiovascular Science. It’s in Baltimore, Md.
One clock is that zap (or action potential). After each zap, the membranes of cells in the SA node must reset. To do this, the body sends ions back across each cell’s membrane to their starting side. The SA node’s zaps can speed up or slow down. But their pace is limited by the membrane’s reset time — which ranges from about 0.1 to 0.5 seconds.
The main ions in an action potential are sodium and potassium. But in the heart, calcium ions also play a timekeeping role, Lakatta notes. Normally, pacemaker cells store positive calcium ions deep inside themselves. But some of these positively charged ions are constantly dribbling out into the body of the cell.
When the number of these escapees gets high enough, the cell membrane’s ion channels open. This triggers an action potential (mentioned above). Later, the body will send those calcium ions back into storage. The length of time this takes serves as the second sort of clock. Put together, the two clocks keep your SA node generating new electrical zaps all on its own — and make sure it keeps them at a healthy pace.
Do you have a science question? We can help!
Submit your question here, and we might answer it an upcoming issue of Science News Explores
When the pacemaker keeps bad time
Unfortunately, things sometimes go awry. Cells in the SA node might not fire together. Sometimes they might not fire at all. In those cases, doctors might install a battery-powered device to serve as the master pacemaker. It sends timed micro-zaps to the SA node to keep it running.
If that node intermittently stops, then the heart will need a special pacemaker, Lakatta says. This one “doesn’t work unless it’s needed.” It can sense how fast the SA node is working and only kicks in when the that node is working too slowly. That’s why it’s known as an on-demand pacemaker, he says. “You know, like you can get movies on demand.”
But the pacing of heartbeats isn’t behind all heart problems. Sometimes the vessels that move blood around get blocked. Lacking blood and oxygen, a spot of cells in the heart tissue may die. This is known as a heart attack.
Although many people can survive a heart attack, this event leaves a scar. Here, the heart no longer conducts or responds to electrical signals, which can upset its stable beating.
Ren-Ke Li is a cardiovascular surgeon at the Toronto General Hospital Research Institute in Canada. He and his team have developed a tiny patch for heart-attack survivors. Made of a hydrogel, this patch can conduct pacemaking zaps across scarred heart tissue so that the heart’s healthy cells won’t stop contracting in unison.
His team developed a second hydrogel, too. It reinforces the heart’s electrical timekeeping. This pair of gels also provides a scaffold on which new heart cells can cling and grow.
Right now, Li’s group is testing these gels in rats. Someday, the patches might help human hearts heal, all the while keeping their electricity flowing.
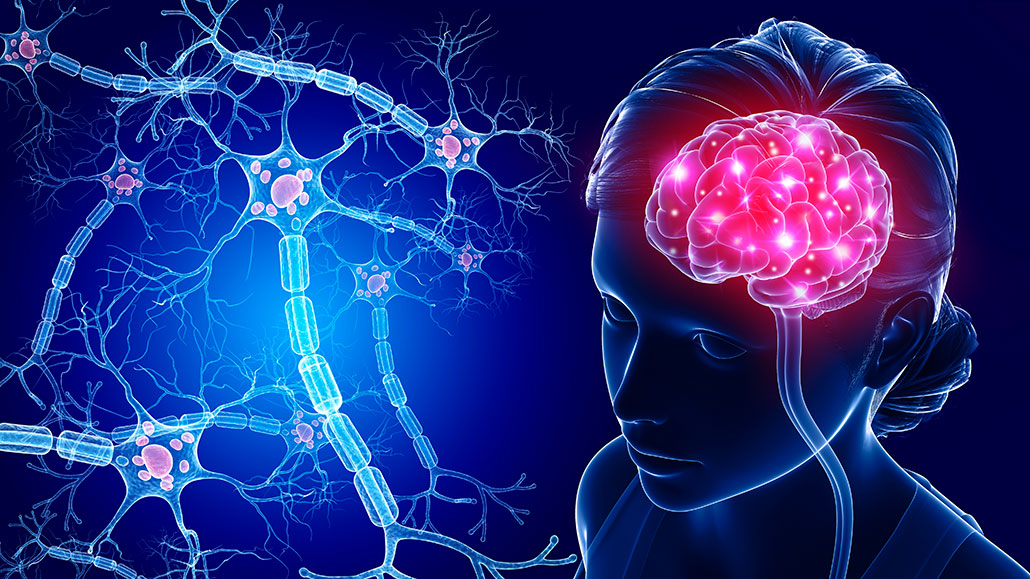
Electricity on the brain
The brain is another hive of electrical activity. Some of its cells — neurons — have long tails, called axons. These serve as paths along which electrical signals travel. Those electrical signals are how the brain processes and transmits information.
Like pacemaker cells in the heart, neurons are more negatively charged on the inside than on the outside. When an electric signal needs to get passed between neurons, ion channels open up in the cell’s membrane. As with heart cells, this allows ions to flow in and out — changing the neuron’s electrical charge.
Neural signals move fast. A signal can sprint from the nerve cells in your big toe to those in your spinal cord and on up into your brain at up to 120 meters (400 feet) per second. So if you’ve stubbed your toe, you’ll know in less than half a second. And in another half second, signals from the brain back to the leg and toe will help you hop around in pain.
It’s not just one neuron that sends a signal from the toe to the brain and back. Groups of brain neurons fire together in what’s known as oscillations, notes Helen Mayberg. She’s a neurologist — someone who studies and treats diseases of the brain. She works at the Icahn School of Medicine. It’s part of the Mount Sinai Health System in New York City.
Each oscillation is the sum of all the firing by neurons in a given area. Mayberg likens it to a choir of cells singing together. Some cells in this chorus have special bands of insulating material wrapped around their axons (or tails). Called a myelin sheath, each of these bands helps keep ions from escaping so that the electrical melody won’t diminish.
Tiny gaps separate these sheaths from each other along the length of an axon — giving each its own personal space. The uncovered gaps between the myelin sheaths on a neuron’s axon are super sensitive to electrical changes. They also have a lot of ion channels.
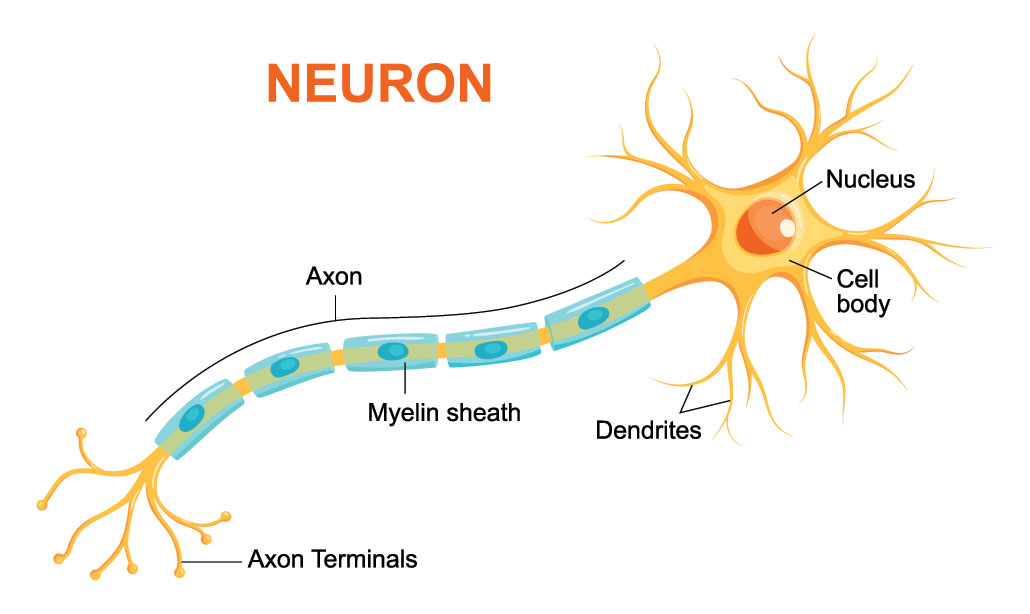
An electrical signal generated in the neuron can skip across these gaps much faster than if it could only ripple down the distance inside the axon. And it bounces along the gaps in the myelin without the signal getting any weaker. This allows the electrical signals to move at the whip-fast speeds needed to let you know that a stove is hot or you stubbed your toe.
When the axons of many neurons in the brain or spinal cord are covered in myelin, they look like a white band. Those bands are electrical superhighways relaying the choir’s tune, explains Mayberg.
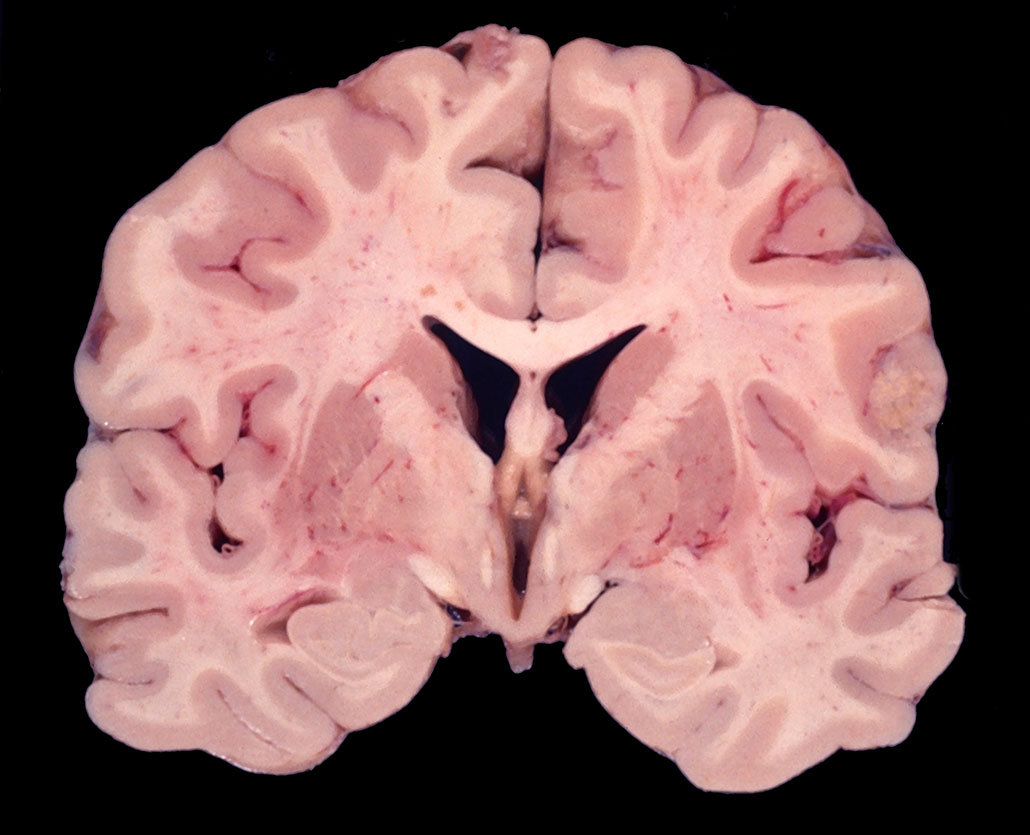
In some brain disorders and diseases, however, something can block the flow of this melody from getting to its intended audience. Perhaps the neurons and their myelin are damaged. Or the cells producing oscillations might get out of sync. Some groups of neurons might even die.
In all these cases, the results can be terrible.
When some neurons in the brain die, for instance, others can start to oscillate out of sync. The body can now get conflicting signals. For instance, it may no longer know when to start a movement. This might cause someone’s limbs to tremble or they might struggle to walk, reach for things and more. These are symptoms, for instance, of Parkinson’s disease.
Brain-zapping therapy
Doctors will sometimes implant electrodes deep into the brain to treat Parkinson’s disease. High-frequency electrical signals from those electrodes will cut off the problem oscillations, Mayberg explains. Now patients can move normally again, at least for a few years.
Mayberg and her colleagues have begun developing a similar deep-brain stimulation for people with depression. This mental illness can trigger extreme, long-term sadness. Medicines or other treatments can help some people with depression. But other people don’t respond well to those therapies.
Mayberg’s group suspected that patients’ resistance to treatment might be due to faulty electrical oscillations in their brains.
To test this, her team sent electrical signals through electrodes implanted at a site right behind the bridge of the nose. This lifted the mood of some patients. Last year, the team had even more success by stimulating nearby white matter (myelin-sheathed neurons).
Before they insert an electrode, Mayberg’s group now studies careful maps of a patient’s brain — and recordings of its electrical activity. “You’re listening,” Mayberg explains, for sites of abnormal cell firing.
“When you find the right spot,” she says, and “inject the current, you interrupt [that abnormal firing] immediately.” Mayberg’s team is finding that a little electricity in the right place can make a big difference in boosting the patient’s mood.
Shocked to the bone
Bones also respond to electricity. Many people think that bone “is sort of inert,” says Greg Wohl of McMaster University. It’s in Hamilton, Ontario, Canada. Inert things are passive. So if bone were inert, it would just passively hold us up and serve as a place for muscles to attach. But bone tissue can adapt, or change, a lot. Sensing electricity is key to that, Wohl says. A biomechanical engineer, he studies how bones respond to physical stress.
And our bones are constantly under stress. It comes from carrying a heavy load.
You put a mechanical load on your skeleton every time you lift a big backpack, do a handstand — even walk. With each stress, Wohl notes, “little cracks are forming and progressing through the bone.”
Over time, those little cracks build up. The same thing happens to a lot of other hard structural materials, he says. Think about the axles on rail cars, the wings on planes — even the bridges over which we drive our cars. With time, the stress of those heavy loads can wear down the support materials. Eventually, Wohl notes, they’ll “need to be repaired by ripping them down or taking them out and replacing them.”
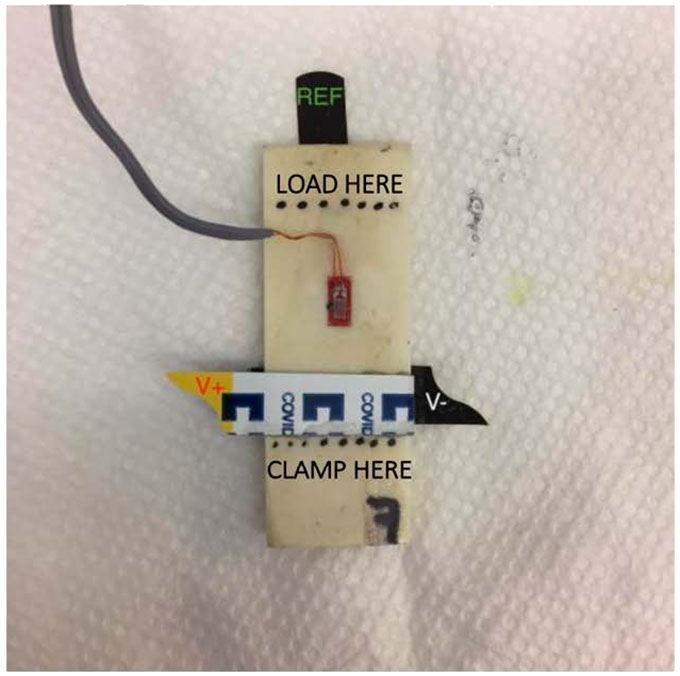
But the body can’t pop in a whole new bone when one wears out. What it can do is break down and replace affected cells around those cracks.
Bone is made up of three main cell types. The first — osteoclasts — are bone munchers. They break down old, worn-down bone bits and re-absorb them. A second type — osteoblasts — help build new bone material where it’s needed. In time, this second type will morph into the third type, true bone cells — osteocytes.
In the 1950s, scientists realized that the surface of bone stressed by weight has a slightly negative charge. If the bone bends slightly (not enough to break), it develops a slightly positive charge. When carrying no weight, the bone has no charge.
This means bone is piezoelectric (PEE-zoh-ee-LEK-trik). It produces an electric charge when bent or squeezed. Wohl has seen this in beef bone that his team got from a grocery store. (“They just gave it to us,” he recalls, “because of course they don’t use it.”)
After cutting it up into little domino shapes, his team put electrodes on each side of those bone pieces. “When we bent [the bone dominoes],” he says, “we were able to generate a little spike of charge that slowly dropped off over time.”
It’s those charges that stimulate bone to make repairs and get stronger. A loaded bone with negative charge stimulates osteoblasts to make more bone. A bent bone with positive charge stimulates osteoclasts to break bone down.
Wohl’s team is now investigating which types of electrical pulses might work best to help boost bone healing.
There are lots of devices on the internet that claim to electrically heal or strengthen bone. But it’s not clear which types will work best, if at all, for a particular type of injury, Wohl says.
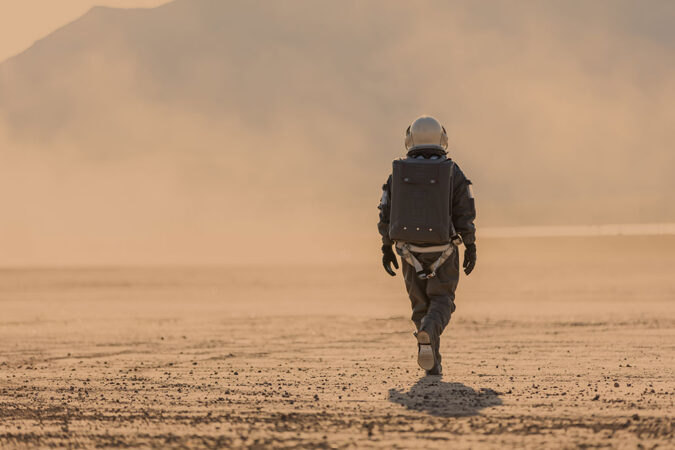
Scientists are also working out how to use those electrical charges to keep bones strong, even when there’s no load on our skeleton — such as during spaceflight. “It’s actually really important, because if we’re going to send people to Mars, they’re going to be in a spaceship,” he says. In that spaceship, they’ll be weightless for a year and a half. During that time, the astronauts’ bones won’t feel a need to repair themselves.
The risk, Wohl says, is that astronauts may “step on to Mars and break a hip.”
All those electrical signals that move through our bodies aren’t just quirks. We couldn’t live without them. Our hearts wouldn’t beat, our brains wouldn’t function and our bones would break. But with just the right jolts of electricity, scientists may be able to lift people’s moods, help their hearts beat in sync and even keep space travelers strong.