Explainer: Telescopes see light — and sometimes ancient history
What they detect depends upon the wavelengths to which their ‘eyes’ are sensitive
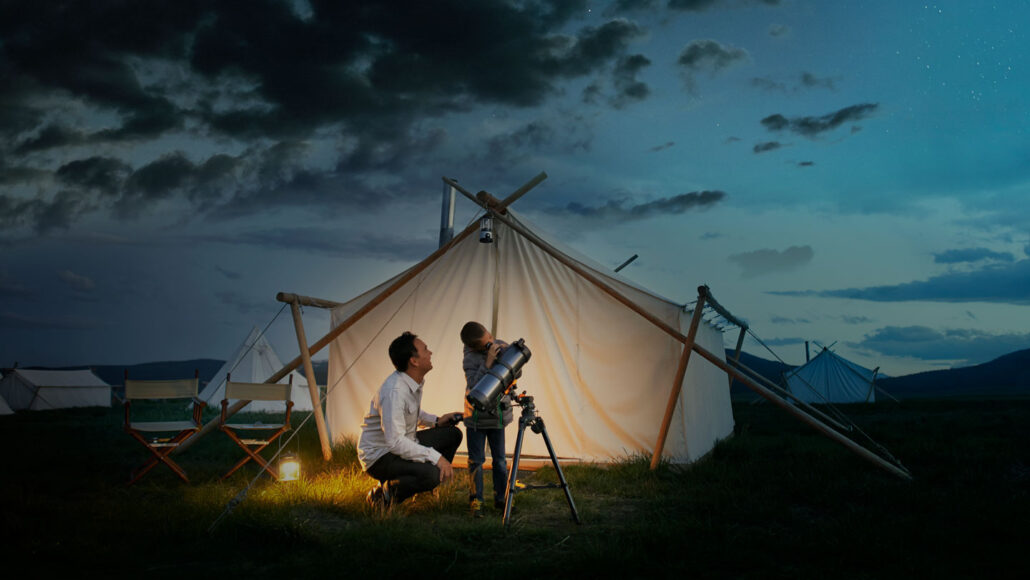
Telescopes can detect light that is fainter than our eyes can see. Some can even see sections of the light spectrum totally invisible to our eyes.
Dana Neibert/The Image Bank/Getty Images Plus
By Trisha Muro
When you look up at the night sky, how many stars can you see? In a big city, you can probably see the moon and just a handful of bright stars. On a cloudless night in a small town or out in the country, you may see thousands of stars. Have you ever looked through a telescope to see these celestial objects even more clearly?
A telescope uses lenses, mirrors or both to focus incoming light from very far away sources. A backyard telescope can easily see craters on the moon or the rings around Saturn. Larger telescopes can show Jupiter’s bands of clouds. Far bigger instruments can peer much, much deeper into the heavens. But how?
It might seem like a telescope is just a big magnifying glass. But there’s more to it than that. A telescope also collects additional light that’s too faint to be seen. You can think of this like setting a bucket outside during a rainstorm. Just as a bigger bucket will collect more raindrops, a bigger telescope will collect more light. Since most objects in space are very far away, their light is extremely dim by the time it reaches Earth. Telescopes help by gathering more light than the unaided eye could ever see.
Very large telescopes are often housed in observatories. Some observatories house multiple telescopes. These buildings are dome-shaped and painted white or silver to reflect sunlight. This keeps the building as cool as possible. The dome protects the instrument from wind, dust and precipitation. Each dome has a large door that rolls open so the telescope can peer out. Both the dome and its telescope rotate so that all parts of the night sky can be observed.
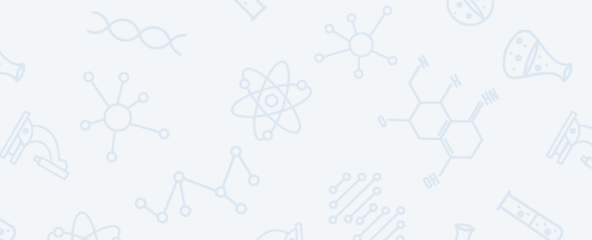
Educators and Parents, Sign Up for The Cheat Sheet
Weekly updates to help you use Science News Explores in the learning environment
Thank you for signing up!
There was a problem signing you up.
It makes sense to build large telescopes away from cities and their light pollution. It also helps to build telescopes high up, such as on mountaintops. That limits how much Earth’s atmosphere can blur the telescope’s views of space.
For a telescope, peering up through the atmosphere is like looking toward the surface of a swimming pool when you’re underwater. Everything looks blurry and wavy, even when the water is still and clear. The lowest layer of Earth’s atmosphere — the troposphere — is to blame. Heat causes ripples in the air. That blurs light from stars, galaxies and planets. Water vapor also smears the light. Dust and pollution cloud the air. City lights also reflect off of molecules in the air, making it harder to see dim stars. Getting above as much of the atmosphere and pollution as possible reduces those effects.
Some telescopes use glass lenses to focus light from space. These are called refracting telescopes. Many other telescopes, known as reflectors, focus light with curved mirrors.
Reflecting versus Refracting
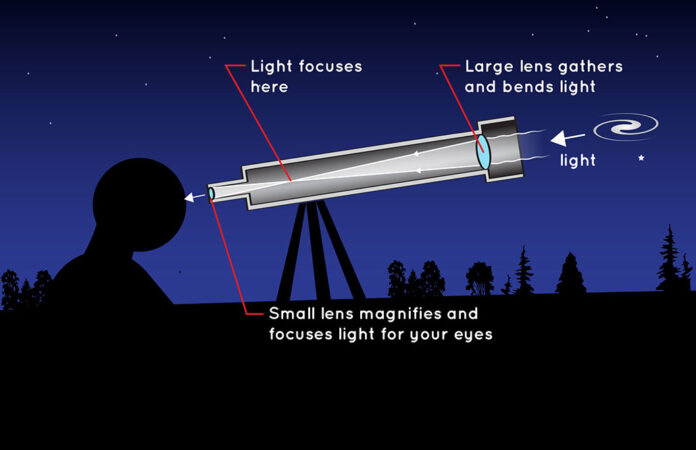
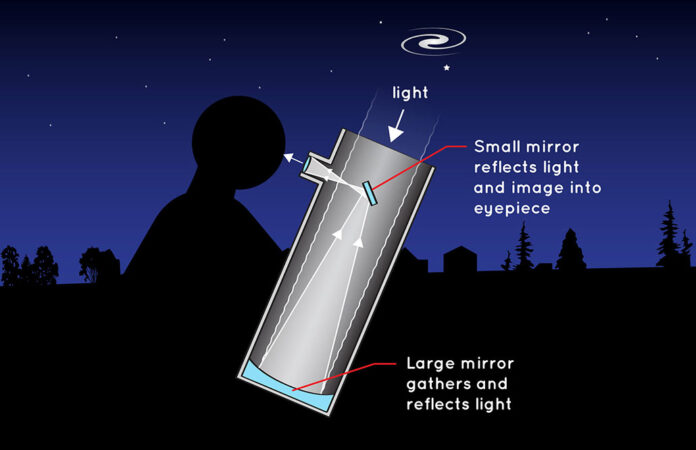
Even when looking through telescopes, however, our eyes are not very good at seeing extremely faint light. Astronomers started using photographic film to capture light from such faint objects more than a century ago. By exposing a telescope to faint light from space for a very long time, it’s possible to collect enough light to show up on film. Now, telescopes are equipped with devices called photomultipliers. If you think back to the example of putting a bucket outside during a rainstorm, these photomultipliers are like buckets that are so sensitive they can capture — and record — a single raindrop in a light drizzle.
When telescopes look at more distant objects, they are also looking farther back in time. The reason: The light that telescopes collect has taken a long time to get here. The farther away a light-emitting object is, the longer the light’s journey to Earth. In some cases, it took billions of years. So the image seen through a telescope now actually reveals how an object looked long ago, when the light was first emitted. In some cases, the source of that light may now be long gone.
Over the last few decades, astronomers have begun sending telescopes into space aboard orbiting spacecraft. That way, there’s no atmosphere to worry about at all. The Hubble Space Telescope is probably the most famous of these space-based telescopes. Launched in 1990, it’s still working today. Hubble collects many of the same wavelengths of light that our eyes can see. This range covers the rainbow, from longer, red wavelengths of light to shorter, violet wavelengths of light.
And there are many more wavelengths of light than what our eyes perceive. Some telescopes focus on these other parts of the light spectrum. In general, imperfections in the smoothness of a telescope’s observing surface need to be smaller than the wavelength it’s trying to detect. Since we can’t see these wavelengths, computer programs color-code telescope images to depict what the telescope sees in ways we can understand. Shorter wavelengths might be depicted in purple, for instance, and longer wavelengths shown in red. Each invisible wavelength that’s detected is assigned a color we can see. The resulting image looks like the real object, just in a “false” or shifted color.
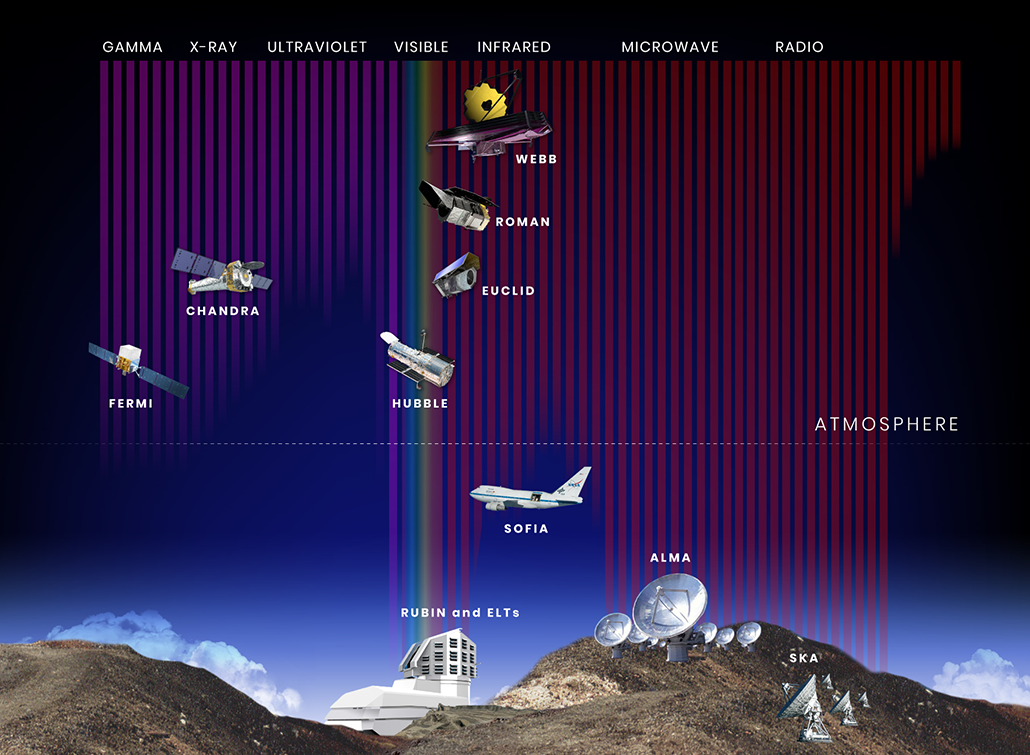
Gathering light longer than the eye can see
Infrared telescopes image wavelengths that are a bit longer than red light. Humans can’t see this light, but we feel some of it as heat. (Have you ever walked past the embers of a campfire that’s no longer giving off light, but still feels warm? That heat energy is carried to your skin by infrared waves.)
Ground-based telescopes can’t pick up infrared light from space. Water vapor in the air absorbs most of it before it reaches the instruments. That vapor is in the troposphere, near Earth’s surface. To avoid this problem, airplanes are sometimes used as infrared observatories. Flying an infrared telescope above this lower layer of the atmosphere allows it to pick up infrared light from space. Planets like Jupiter glow brightly in infrared. Cool stars do, too.
Because infrared light can pass through clouds of dust in space, infrared telescopes can see behind the dust that would obscure other telescopes’ views.


Both images: NASA; ESA; Hubble Heritage TeamLeft: NASA; ESA; Hubble Heritage TeamRight: NASA; ESA; Hubble Heritage Team
Launched on December 25, 2021, the James Webb Space Telescope has been designed to see infrared light from the deepest parts of space. To do that, it has to stay super cold. Otherwise, its own heat would interfere with its observations. In order to stay cool, its orbit will be 1.6 million kilometers (1 million miles) above Earth. That’s four times farther than the moon! This telescope will see planets in other solar systems and the formation of distant stars. It might even glimpse the first galaxies that formed after the Big Bang.
Microwave telescopes view the same type of energy that heats food in a microwave oven. After the Big Bang, the entire universe began to cool. Radiation left over from the Big Bang has been cooling for so long that it has very low energy. Lower energy light has longer wavelengths. So this light has even longer wavelengths than infrared light; they’re in the microwave part of the spectrum. As with infrared light, you need a space-based instrument to see these cosmic remnants of the Big Bang.
A space telescope called COBE — the Cosmic Background Explorer — measured that heat, just as a thermometer measures your body’s temperature. COBE found this cosmic microwave background in our universe is just 3 kelvins. That’s negative 270° Celsius (–450° Fahrenheit)! Astronomers can look at tiny ripples in those temperature measurements to map when and where the very first galaxies started to form.
Radio telescopes pick up wavelengths longer than infrared. (You might think that radio waves are sound waves. In fact, they are a form of light.) Spanning a huge range, radio wavelengths can be as wide as a mountain or as small as a few inches. There are lots of radio telescopes on Earth. Since radio waves are so long, radio telescopes do not have to collect them using smooth, polished surfaces like lenses or mirrors. In fact, many radio telescopes look more like wire mesh. The former Arecibo telescope in Puerto Rico was an especially good example of how rough the surface of a radio telescope can be.
You might have seen pictures of networks of these dish-shaped telescopes, such as the Very Large Array in New Mexico and the Atacama Large Millimeter/submillimeter Array, or ALMA, in northern Chile. Astronomers have learned to use powerful math techniques to link these networks of telescopes together. Such networks can then mimic one super-big instrument. The Event Horizon Telescope is a network of 10 radio observatories across the entire planet. In 2019, it generated the first image of a black hole.
-
The Very Large Array in New Mexico is a network of radio telescopes which are linked together. Mark Newman/Getty Images Plus/The Image Bank/Getty Images Plus -
The Hubble Space Telescope has been in orbit around Earth since 1990 and continues to capture beautiful images in visible wavelengths. NASA -
Kitt Peak National Observatory in Arizona is home to many telescopes of varying sizes. Bryan Allan/The Image Bank/Getty Images Plus
Collecting light shorter than the eye can see
Many wavelengths are far shorter — and higher in energy — than visible light. To picture why shorter wavelengths have higher energies, imagine holding a rope that is tied to something at the opposite end. It’s not very hard to slowly shake your end of the rope up and down. That low-energy motion creates long waves in the rope. If you shake the rope as fast as you can, that requires more energy. As a result, the waves in the rope get much closer together.
Ultraviolet (UV) telescopes focus on light whose wavelengths are just a bit shorter than violet light. You may have heard about this kind of light in terms of sunburn. Earth’s atmosphere blocks some of the sun’s UV rays. Still, much gets through. For astronomers, UV light can reveal stars hotter than our sun. Many young stars shine brightly in UV light, allowing us to learn a lot about how they form inside galaxies. UV waves can also be created when the shock wave of an exploding star, or supernova, slams into a cloud of dust or gas.
X-Ray telescopes visualize the same light that doctors use to see a broken bone. X-rays are shorter than both visible and UV light. In space, these wavelengths point to very high-energy objects, such as black holes and supernovas. On December 9, 2021, NASA launched a new X-ray telescope. Called IXPE — for Imaging X-ray Polarimetry Explorer — it will explore how magnetic fields can shape and steer an object’s X-ray light.
Gamma-rays are the highest-energy wavelengths in existence. So gamma-ray telescopes point to extremes in space. Many cosmic sources of gamma rays remain a mystery. It’s up to scientists to explain where they come from. The only gamma-ray telescopes are in space. Sometimes they detect a sudden flare, called a gamma-ray burst. When this happens, telescopes of all kinds swing into action to see if the burst appears in visible or other wavelengths, too. That could help astronomers figure out the source of these strange, super-powerful bursts.
In their many forms, telescopes reveal details of objects in space that are invisible to our eyes. They help us to see farther. And they capture light that is too dim to otherwise notice. Just as color photographs show more than black-and-white, telescopes of all wavelengths reveal details of the cosmos that our unaided eyes could never see.