Batteries should not burst into flames
Exploding devices have prompted work on lithium-ion batteries that won’t burn
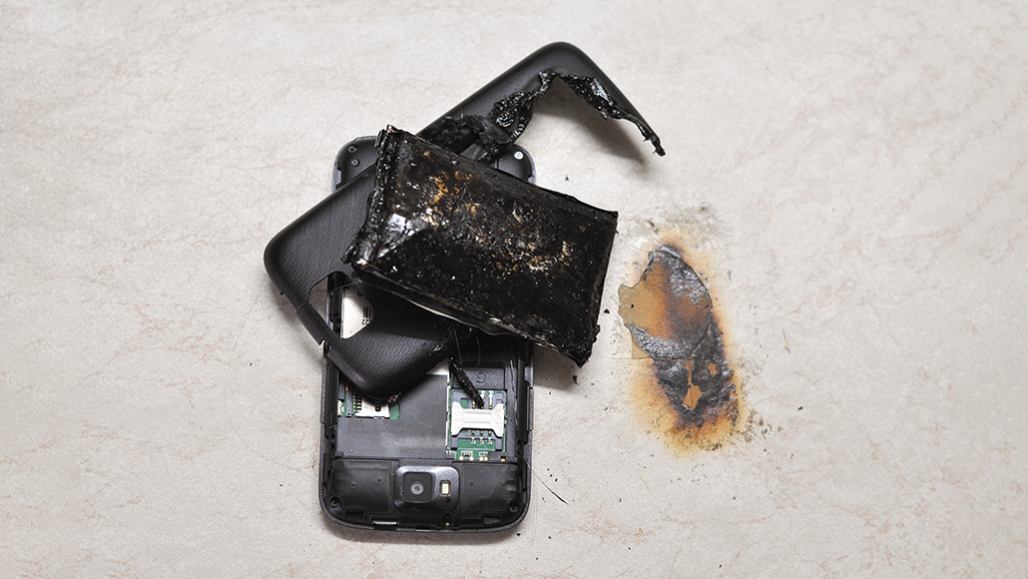
Overheating caused the battery in this cellphone to explode and burn.
Ivan Marjanovic/iStock/Getty Images Plus
The Mahoneys’ hoverboard turned out to be a blast from the past. But not in a way the Stoneham, Mass., family had hoped.
The toy’s wheeled platform can carry a standing rider around the neighborhood. This one had sat unused for years. A few last spins before donating it to charity seemed like fun. So mom plugged it in to charge its lithium-ion battery.
While charging, the battery overheated and exploded. The ensuing flames set the family’s house on fire. A teenage daughter was home at the time. As the house filled with smoke, she climbed out a second-story window and onto an overhang. From there, she jumped to the ground as police officers stood by. The 2019 episode caused hundreds of thousands of dollars’ worth of damage, according to news reports.
Chemist Judith Jeevarajan has heard a lot about problems with products powered by lithium-ion batteries. She studies battery chemistry and safety for Underwriters Laboratories in Houston, Texas. The company carries out safety research on products that we use daily.
In the United States alone, a government safety agency has received thousands of reported failures by lithium-ion batteries. The good news: Rates of catastrophic failures have fallen, Jeevarajan says. Today, perhaps 1 in 10 million lithium-ion batteries fail, she says. And reports of hoverboards catching flame have waned. Now Jeevarajan hears more about problems with the batteries in e-cigarettes.
This includes a 2018 vape-pen explosion that sent a teen to the hospital with a shattered jawbone and a hole in his chin. One study estimates that between 2015 and 2017, more than 2,000 battery explosions or burn injuries sent vapers to the hospital. There were even a couple of deaths.
The problem is that an overheated e-cig battery can get out of control fast. Users may be hurt badly, Jeevarajan says. “But then also … the carpet’s burning, the drapes are burning, the furniture is burning and so on.” Despite having just one lithium-ion cell in it, she notes, a failed e-cig battery “can cause so much damage.”
Fortunately, most lithium-ion batteries work as intended — and don’t catch fire. But when one does, the result can be catastrophic. So researchers are working to make these batteries safer while engineering them to be even more powerful.
Lithium-ion revolution
Lithium-ion batteries are everywhere. They’re in cell phones, laptop computers and even toys. Tiny ones power wearable electronics. These batteries “have really revolutionized our world,” says Neil Dasgupta. He is a mechanical engineer at the University of Michigan in Ann Arbor. Some automakers are starting to replace gasoline engines with lithium-ion batteries. That could allow us to use renewable energy resources to fuel our cars, Dasgupta notes.
The technology is such a big deal that the scientists who made key advances took home the 2019 Nobel Prize in chemistry.
Lithium-ion batteries made their debut in consumer electronics in 1991. They were bulky and didn’t provide much energy. Since then, they’ve gotten smaller and cheaper and hold more energy. But there’s still room for improvement. One of the big challenges, Dasgupta says, is increasing energy storage without sacrificing low cost or safety.
Scientists usually describe energy storage as the total energy divided by a battery’s weight or volume. This is a battery’s energy density. If scientists can increase this density, then they can make smaller batteries that still provide lots of energy. This could make for lighter laptops, for instance. Or electric cars that travel farther on a single charge.
Energy density is one reason lithium is so attractive to battery-makers. The third element of the periodic table, lithium is super lightweight. Using it helps to pack a lot of energy into a small or lightweight unit.
Batteries make an electric current through chemical reactions. These reactions occur at the batteries’ electrodes. The anode (AN-oad) is the negatively charged electrode when the battery is supplying power. The cathode (KATH-oad) is the positively charged one. Ions — molecules that have a charge — move between these electrodes in a material called an electrolyte.
Anatomy of a lithium-ion battery
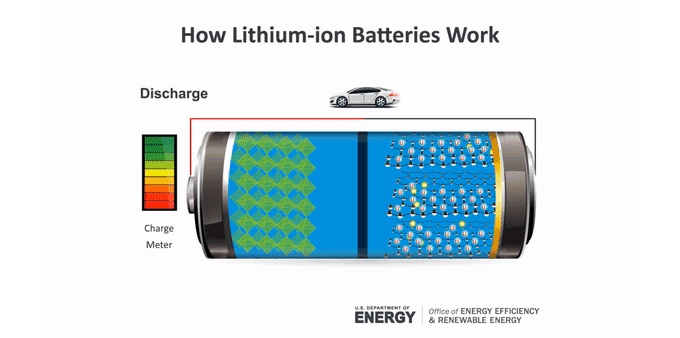
Inside a battery are two electrodes where chemical reactions occur. Those reactions create charges that let the battery provide an electric current.
In a lithium-ion battery, lithium atoms at the anode split. This makes electrons and lithium ions (lithium atoms with a positive charge). The lithium ions move within the battery to the cathode through an electrolyte. Electrons generally can’t pass through this material. So the electrons take a different path to the cathode through an external circuit. That creates an electric current that can power a device. At the cathode, the electrons meet up with the lithium ions for another chemical reaction.
To charge a battery, this process runs in reverse. The ions and electrons journey back to the anode. In a lithium-ion battery, that anode usually is graphite. The lithium ions tuck between the atom-thin layers of the graphite. The cathode can be one of several lithium-containing materials.
That electrolyte makes lithium-ion batteries a potential fire hazard. The electrolyte is a flammable, carbon-based (organic) liquid. Organic compounds allow lithium-ion batteries to reach high voltages. That means the battery can store more energy. But these organic electrolytes can fuel a fire if the battery overheats.
Such overheated batteries have caused fires and worse — explosions.
Thermal runaway
A lithium-ion battery can overheat if it has too much or too little charge. Battery designers use a computer chip to control the charge level. When your device’s battery is reading 5 percent, it’s not almost entirely out of juice. But if the battery were to discharge way more, or be charged up too much, dangerous chemical reactions could occur.
One of these reactions forms lithium metal on the anode (instead of storing lithium ions inside the anode). “That can actually cause hotspots. And [the metal] can react with the electrolyte,” Jeevarajan explains. Another reaction releases oxygen gas from the cathode. With heat and a flammable electrolyte, she says, this is “a really good combination to [start] a fire.”
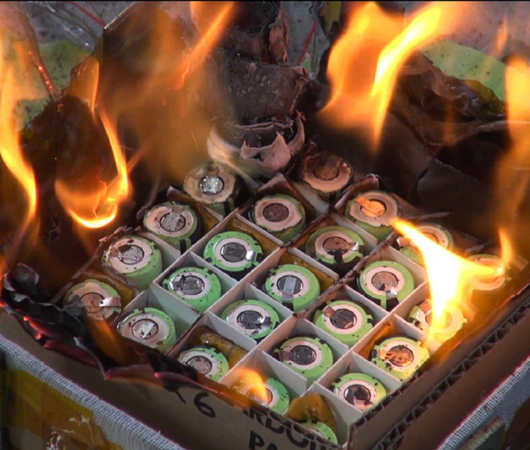
This can spark a process called thermal runaway. “These things [can] happen so rapidly, that it’s very uncontrollable,” Jeevarajan says. Those heat-producing reactions fuel themselves. They become hotter and hotter. A runaway pack containing many batteries can quickly reach more than 1,000° Celsius (1,832° Fahrenheit).
Physical damage can also cause heat-producing reactions. A separator keeps the two electrodes apart. But if something crushes or punctures a battery, they can touch. That would cause them to react, producing a rush of electrons. This is called a short circuit. It can release a lot of heat and set off thermal runaway.
So some engineers are working to make batteries less likely to catch fire in the first place.
Solid-state of mind
Replacing the flammable liquid in lithium-ion batteries would tame their risk of flame. So engineers such as Dasgupta and his team in Ann Arbor are looking into solid electrolytes.
One type of solid electrolyte employs polymers. These are compounds like those used to make plastics. Dasgupta’s team is also working with ceramics. These materials are similar to what some dinner plates and floor tiles are made from. Ceramic materials are not very flammable. “We can put them into the oven at very high temperatures,” he notes. “And they’re not going to catch fire.”
Solid electrolytes might be safer, but they present new challenges. An electrolyte’s job is to shuttle ions around. This is generally easier and speedier in a liquid. But some solids would let lithium zoom through almost as well as in a liquid.
Batteries that use such solid electrolytes still need more work. Engineers are trying to figure out how to boost their performance and manufacture them more reliably. One problem that Dasgupta and his team are tackling: forces inside such batteries. Forces are created at the site where a solid electrolyte makes contact with a solid electrode. These forces can damage the battery.
To make a more powerful battery, Dasgupta’s team and others are looking to change the anode. Graphite — the same material as pencil “lead” — is a typical anode material. It acts like a sponge for lithium ions. The downside is that it limits how much energy a battery can hold. By replacing a graphite anode with lithium metal, the battery might be able to hold five to 10 times more charge.
But lithium metal has its own problems.
Remember how scientists don’t want to let lithium metal form on a battery’s anode? That’s because “it’s a very reactive material,” explains Dasgupta. “Lithium metal reacts with almost everything.” (Drop a piece in water, for instance, and it creates a bright pink liquid bubbling with gas.) It’s even hard to keep lithium from reacting with a battery’s electrolyte, he notes.
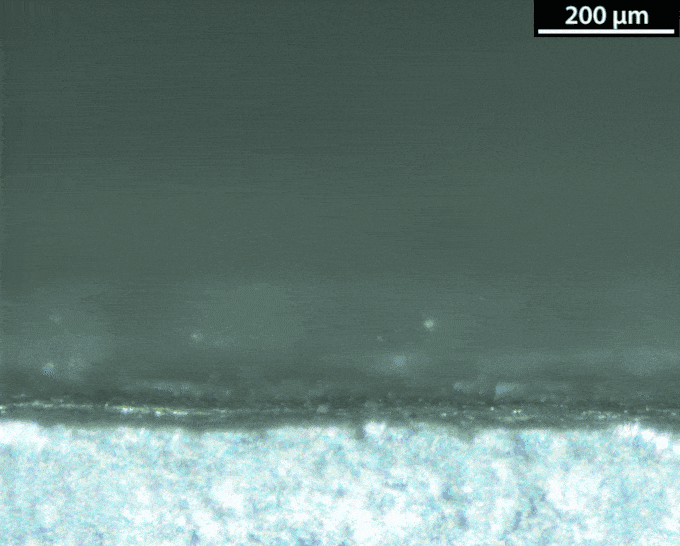
With a lithium-metal anode, the battery would be doing the thing avoided in normal lithium-ion batteries: making metallic lithium during its recharge. That’s not a smooth process. Instead of forming a nice flat surface, the new metal takes on interesting shapes — mossy structures called dendrites. Those dendrites can pose dangers. They can stab the separator that keeps the anode and the cathode apart. And that risks leading to a short circuit and thermal runaway.
Dasgupta and his team figured out how to watch those dendrites grow. They made a battery and hooked it up to a microscope. The anode surface is super important, they learned. Most surfaces aren’t perfectly smooth. They have defects, Dasgupta notes. These include impurities and sites where the atoms have shifted.
A defect can turn into a hotspot. “When you try to charge the battery, now the lithium ions really like to focus in on this hotspot,” he says. Hotspots are where dendrites tend to start growing. To prevent dendrites from forming, the group is engineering the surface at the nanoscale. Instead of making the surface super flat, they could maybe shape it in a way that controls hotspots.
A battery that won’t go up in flames
Spencer Langevin holds a blowtorch to a coin-sized battery electrolyte. Under its roughly 1,800 °C (3,272 °F) temperature tip, a layer of gel crackles like the caramel crust on the fancy-pants dessert, crème brûlée (Krem Bru-LAY).
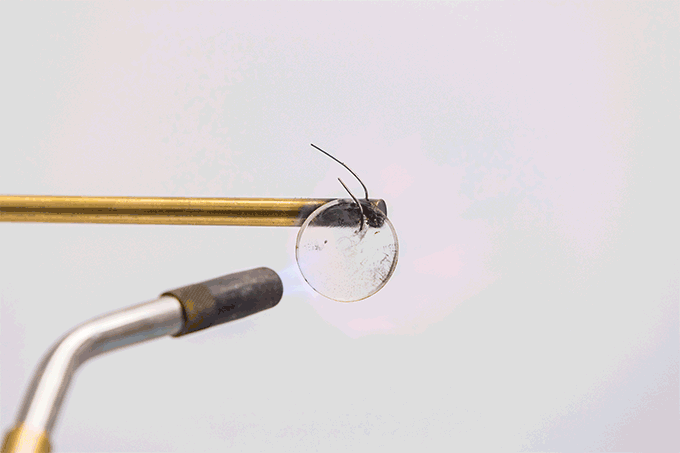
That sound is water in the electrolyte boiling, the chemist explains. Langevin is part of a team that made the electrolyte. They work at the Johns Hopkins University Applied Physics Laboratory in Laurel, Md. The electrolyte material glows rocket red. That’s because of the lithium it contains. But this material does not burst into flame.
Langevin and his team described this novel electrolyte in the November 11, 2019 Chemical Communications.
The torch’s tip is way hotter than temperatures reached in thermal runaway, notes chemist Adam Freeman. He also works at the laboratory in Laurel. If batteries contained this electrolyte, “at least the whole thing won’t act as a fuel source,” he says.
The team has shown that they can cut off the scorched part of the battery and the cell keeps working. Even after being cut, it still puts out enough energy to run a small fan. They’ve sliced up cells. They’ve dunked them in water. They’ve even shot holes through them with an air cannon to simulate gunshots. Not even that firepower made them ignite.
The electrolyte is based on a hydrogel. That’s a type of water-loving polymer. Chemists usually steer clear of water when making batteries. Water limits a battery’s voltage range. If the voltage goes too high or too low, the water itself becomes unstable.
But that doesn’t happen here. The reason is that the polymer latches onto the water. Lithium salts provide the ions that move through the new electrolyte. These components give the electrolyte its name: “water-in-salt.” The water-in-salt material is stable across a fairly broad range of 4.1 volts. That approaches what today’s lithium-ion batteries can provide.
What’s “important is to try to move toward nonflammable electrolytes,” says Stefano Passerini. He’s a chemist in Germany at the Helmholtz Institute Ulm. But, he adds, “this paper doesn’t really demonstrate that it’s possible to use [water-based] electrolytes for high-energy batteries.” One reason: The anode material they used limited the energy density.
In the future: More recharges
One big goal for researchers working with water-in-salt and solid electrolytes is increasing the number of times their batteries can be recharged. Lithium-ion batteries slowly lose their capacity to hold charge. An iPhone battery might be able to charge and discharge some 750 times over several years. Langevin’s team has so far reported only 120 such cycles for a battery with its electrolyte. This group is shooting for one that will work through thousands of cycles.
Everyone would love to have small, lightweight batteries that power their phones longer and last for years. But we can’t forget the occasional battery calamity, such as the one that set the Mahoney family’s home on fire. As engineers and scientists seek to pack more energy into batteries, safety remains a key goal.