Like Magneto? Microcrystals give magnets superpower over living cells
These iron-rich protein crystals could be the future of how scientists study nerve cells
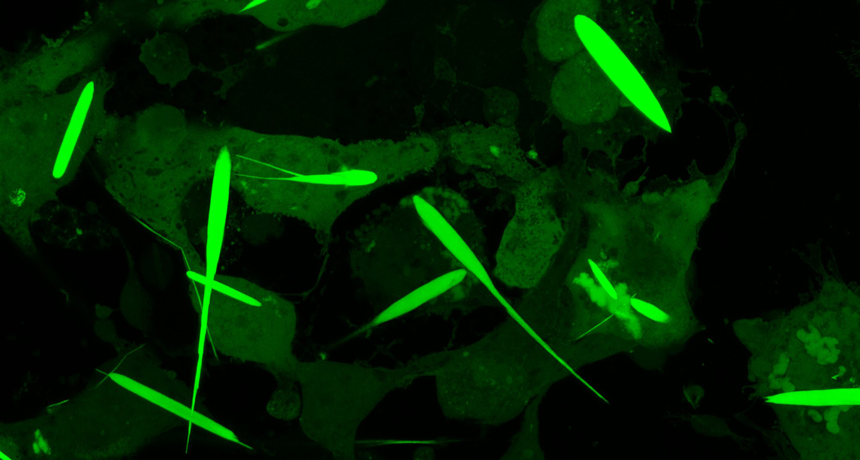
Labeled with a glowing protein that gives them an eerie green glow, these needle-like protein crystals are jammed full of iron. That lets scientists control the crystals — and the cells they’re inside of — with a magnet.
Bianxiao Cui
By Jeremy Rehm
Imagine if you could control someone by using a magnet. It would be a bit like Magneto, the supervillain in X-Men. He can control anything magnetic. Even the iron inside someone’s body.
Controlling people with magnets sounds a little, well, wacky. But scientists have now done something close to that. They have engineered cells to make long, needle-like crystals rich in iron. Researchers can then use magnets to control cells containing these crystals.
Video recordings show these iron-rich crystals moving toward a strong magnet. The crystals pull the entire cell along with them.
“It’s almost alien,” says Bianxiao Cui. She’s a chemist at Stanford University in California.
Cui and her colleagues didn’t set out to give scientists superpowers like Magneto’s. Instead, their new protein crystals were designed to help scientists study which neurons control an animal’s movements and senses. The crystals provide something inside a cell that magnets can attract. This innovation fills a gap in the budding field of magnetogenetics (Mag-NEE-toh-jeh-NET-iks).
Scientists in this field genetically engineer cells so that they will respond to magnetic fields. Now researchers can remotely control specific neurons in the body using magnets. Those neurons could be ones that control how hungry an animal gets. Or they could be neurons that control leg muscles so a mouse starts running when a magnet is nearby.
Gaining magnetic control
A magnetic field can turn on neurons that contain proteins rich in iron. The field does this by heating or giving a mechanical push to those proteins.
Researchers had already been able to control neurons with light. That process is called optogenetics. To use it, scientists insert light-sensitive molecules into the neurons of living animals. The researchers can then turn the neurons on or off simply by shining a light on them. With this technique, neuroscientists have done some incredible things. They’ve made mice run in circles. They’ve even restored movement to an animal’s paralyzed leg.
But optogenetics has its downsides. Light, for example, can’t penetrate deeply into the body. There’s just too much bone, muscle and other tissue in the way. So researchers may implant optical fibers into the animal to deliver light to deep neurons. That makes the method cumbersome and even potentially dangerous.
The whole idea behind magnetogenetics is that you don’t have to implant anything, explains Jacob Robinson, who was not involved in the study. He’s a neuroengineer who works at Rice University in Houston, Texas.
Cells deep inside the body could be switched on with just a magnetic field. No fibers or surgery would be needed.
But there’s a snag. The only protein found naturally inside animal cells that’s even remotely magnetic is ferritin (FAIR-ih-tin). Each molecule can have as many as 4,500 atoms of iron. That may sound like a lot, but it’s not. The force that a magnet acting on ferritin generated would be only a billionth as strong as would be needed to turn on a neuron. So Cui’s team developed protein crystals that could carry enough iron to make their cells responsive to magnets.
Giant crystals with an iron heart
The team first extracted the gene to make ferritin from a microbe. They then made a circular piece of DNA that contained two human genes. Those genes make long, hollow crystals called inka-PAK4 (short for Inkabox-PAK4cat). The team introduced these circular pieces of DNA into human kidney cells that were growing in a petri dish. A day later, the first crystals appeared.
“When I first saw those crystals assemble in the cells by themselves, it was just amazing,” Cui recalls.
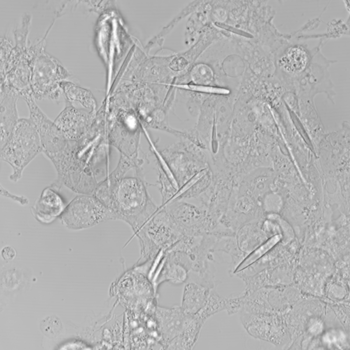
The crystals grew for three days until they were 45 millionths of a meter long. That’s about half the average thickness of a human hair. They’re the largest iron-containing protein crystals ever made in the lab — or in nature, Cui says. They were even longer than the cells they grew in. But the cells in which they formed never ripped. They just stretched to accommodate the crystals.
The researchers pried open the cells and removed the crystals. Then they loaded these with iron. The team estimates that it packed some 8 billion iron atoms into each crystal before inserting those crystals into human cells growing in a dish. Now they exposed the cells to a magnetic field and waited to see what would happen.
And the cells moved.
“The first time I actually saw [the cells] move toward the magnet, I was like, ‘Wow!’” Cui says.
Crystals started collecting close to the magnet. And the crystals pulled their cells with them. The team described this online September 25 in Nano Letters.
Robinson expressed excitement over this. “It’s an excellent step,” he said, “toward engineering cells to create their own magnetic nanoparticles.”
Scientists aren’t sure what will happen to the crystals afterward. But the cells have the genes for the crystals. So every cell reproduced from the original cells should be able to make the crystals, Cui says.
Iron not included
As promising as the results are, both Cui and Robinson emphasized that this isn’t the end.
“We still haven’t reached the goal,” Cui says.
Ideally, researchers would not need to first remove newly grown crystals to pack them full of the metal atoms. Instead, cells would enrich the crystals with iron as it built them. In fact, Cui’s group tried three different ways to get iron into its cells. They even drenched the cells in an iron-rich solution. Nothing worked.
Cells typically keep their iron levels low, Cui’s team notes. It’s estimated that cells naturally contain only 3 percent as much iron as the crystals would need to be effective.
We probably need to alter the cell’s outer membranes, Cui suspects. Then, she says, they might be able to transport more iron into a cell. Still, these magnetic crystals are a major leap forward in the young field of magnetogenetics. And the researchers are confident additional studies will overcome this iron-enrichment obstacle.
This is one in a series presenting news on technology and innovation, made possible with generous support from the Lemelson Foundation.