The war on superbugs
New innovations are exploring how to battle extra-menacing bacteria
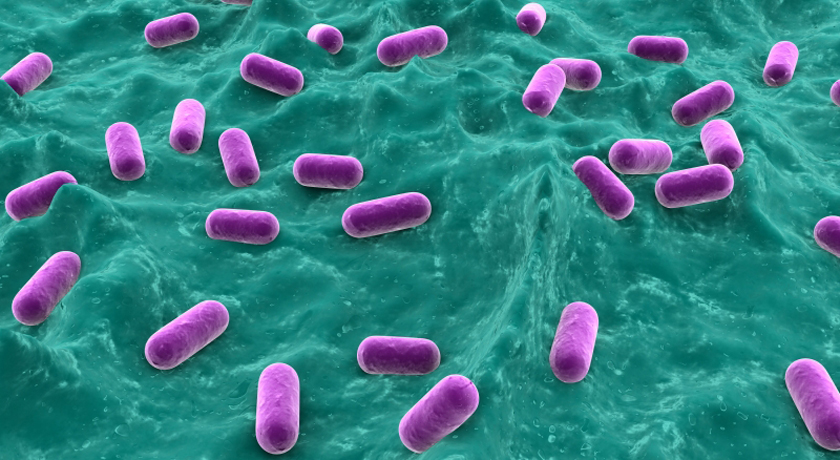
Lactobacillus bacteria, such as those shown here — colored purple to show up against the background tissue — can be tweaked to hunt down and poison disease-causing germs. Think of them as little antibiotic factories.
Eraxion/ iStockphoto
The second of a two-part series: Read part 1
Antibiotics are wonder drugs. They treat a range of bacterial infections, from battle wounds and pneumonia to tuberculosis and pinkeye. But these medicines are losing their edge. Germs are becoming immune to the drugs that had been created to slay them. This dangerous trend has been emerging across the globe.
Called resistance, the phenomenon poses a growing health threat. It occurs naturally. Microbes are constantly acquiring mutations — accidental genetic changes. Those that help them outsmart the medicines allow them to survive.
Consider tuberculosis, or TB. This bacterial disease infects the lungs. It has been killing people — legions of them — for most of recorded history. Today it takes the lives of some 2 million people each year. And across the globe, it remains the leading cause of death among young adults.
Someone with active TB need only talk, cough or sing to spew bacteria into the air. All it takes are a few TB cells to become infected. Today, another person aquires TB every second of every day, according to the U.S. National Institutes of Health.
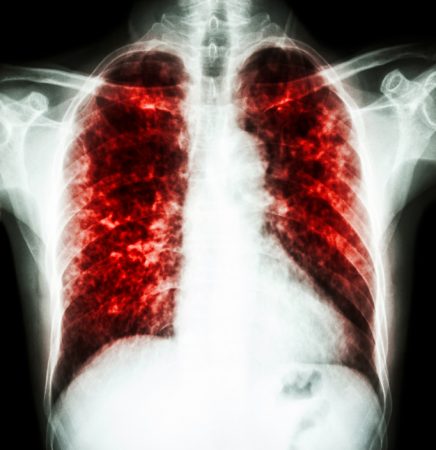
But that isn’t going to happen. A super-TB germ began to surface. It is proving immune to one or more antibiotics. Today, cases of TB that are resistant to two or more antibiotics have shown up in 92 countries. They cause almost a half-million of these multi-drug-resistant infections each year. And because they are hard to treat, it is highly likely an infected person will spread these germs. Some disease experts worry that in the near future, TB may no longer respond to any drug.
Fortunately, as we show here, physicians and scientists are actively working to beat this problem with new policies and treatments.
Getting doctors to prescribe fewer antibiotics
Drug resistance climbs when antibiotics are overprescribed. And a new study in the July 10 Lancet Infectious Diseases points to just how fast the prescriptions for antibiotics have been growing. The new analysis looked at pharmacy sales for these drugs in 71 countries. Worldwide, it found, antibiotic use rose 36 percent over just 10 years: 2000 to 2010. Ramanan Laxminarayan led the research team. He’s an epidemiologist at the Princeton Environmental Institute in New Jersey.
Doctors know that prescribing antibiotics drives up resistance. Yet here’s one reason many of them feel they have to write so many prescriptions. They think: “If I don’t give antibiotics and the patient winds up having an infection, it could be catastrophic” for that patient, says Scott Flanders. He’s a doctor at the University of Michigan in Ann Arbor.
“Physicians view the patient sitting in front of them as their first and foremost responsibility,” he notes. Doctors often prescribe an antibiotic if they believe a person may have a treatable infection. What’s untreatable? A virus: Antibiotics do not work against viruses.
Lately, researchers have been working on a few thoughtful ways to help doctors stop prescribing antibiotics for infections they won’t help.
Daniella Meeker of the RAND Corp. in Santa Monica, Calif., developed one of them. She works with doctors and researchers in southern California. Her team identified 15 doctors around Los Angeles who regularly treat adults. It asked half of these doctors to sign a written pledge. In it, the doctors vowed not to prescribe drugs for any illness that was likely due to a virus.
The signed pledge hung as a poster for 12 weeks in each doctor’s exam room. That way, all patients could see it. The other doctors signed no pledge and hung up no poster. All of the doctors continued seeing patients as usual.
Signing that pledge was a relatively simple thing to do. Yet it had a strikingly big effect.
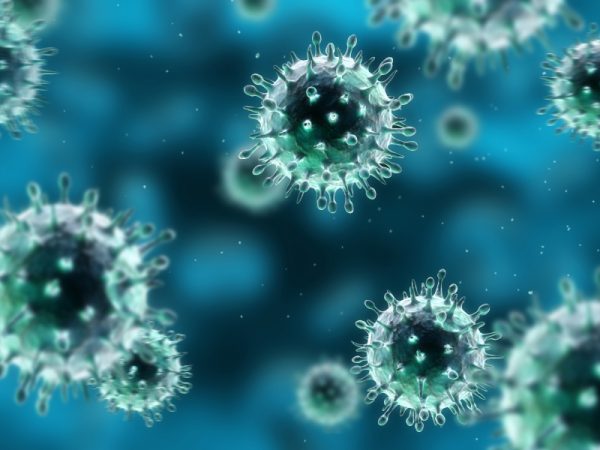
Flu is a viral disease. And antibiotic prescriptions dropped by 20 percent during flu season. Most of the drop seemed to come from no longer prescribing antibiotics for flu. But this drop showed up only among doctors who had signed the pledge. Meeker’s team reported its findings January 27 in JAMA Internal Medicine.
Another strategy makes use of hospitalists. These are doctors who work for a hospital, focusing on the care of admitted patients. Research has shown “it’s safe to stop antibiotics that do not appear to be effective,” Flanders notes. Hospitalists can record when antibiotics are prescribed, when the patient took them and how symptoms are progressing. They also can begin looking for signs — starting a few days after treatment has begun — that a patient may have a virus and therefore not need to continue use of antibiotics.
The CDC has proposed that hospitals routinely follow this kind of practice. A June 2013 report in the journal Clinical Therapeutics found that many hospitals now do this. And it’s been cutting inappropriate prescriptions for antibiotics.
Technology and innovation
But there are times when this approach won’t work. For instance, some diseases such as tuberculosis “take ages to grow” in the lab, Sharon Peacock told Science News for Students. She’s a microbiologist at the University of Cambridge in England. For such germs, it might take two months to figure out which drugs would work best. And doctors can’t wait that long to start treating an infection.
Fortunately, help may be on the way.
Researchers can get a full read-out of all genes in a TB germ in just a day or two. Then they can scout for altered genes that have been linked with resistance to a particular germ-killing drug, Peacock notes in the May 29 Nature.
At a July 11 meeting in Washington, D.C., the President’s Council of Advisors on Science and Technology, or PCAST, also pushed for doctors to use this approach across the United States. Explains PCAST co-chair Eric Landers, three patients may show up the same clinic with the same disease. Right now, it’s impossible to know if their diseases come from the same germs or from slightly different versions — ones that may respond differently to drugs.
However, each bacterium’s DNA “contains a record,” says Landers. He’s a systems biologist at Harvard Medical School in Boston, Mass. If the germs in each patient are identical, which would happen if they spread from one patient to another within a facility, their DNA “will be almost identical.” But If they came from different places, small “telltale genetic differences” will show up in the germ’s genes.
Other researchers are working to enhance how well existing drugs work. James Collins of Boston University is one of them. This bioengineer made use of phages (PHAH-zhez) — viruses that infect bacteria. His is to create phages that can help antibiotics work against even resistant bacteria.
Previously, his lab showed that antibiotics trigger chemical reactions that damage DNA. So his team engineered the creation of new phages. These viruses make proteins that derail the bacteria’s DNA-repair system. (Proteins are the molecules that carry out a cell’s activities.)
The novel phages can boost by 100 to 10,000 times how well an antibiotic works, the researchers reported in 2009. In essence, Collins explains, the phages re-sensitize superbugs. It’s “making them vulnerable to the drug to which they’ve grown resistant,” he says.
Enlisting good bacteria to fight bad ones
Another problem: Doctors often prescribe broad-spectrum antibiotics. These are drugs that kill many different types of germs. But because such medicines aren’t very precise, they also may kill many beneficial bacteria, including those living inside us.
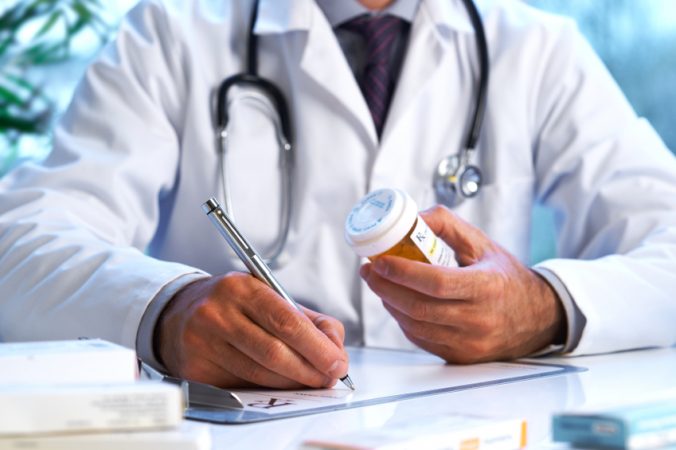
With that in mind, Collins’ team started a new line of experiments. They work with Lactobacillus (LAK-toh-bah-SILL-us). It is the type of good bacteria found in yogurt. Inside the human gut, these helpful bacteria can fight infections.
Collins’ group wants to re-engineer the genes in Lactobacillus. This new breed of bacteria would be able to sense when disease-causing bacteria are nearby. Then it would produce a toxic substance, one tailored to kill only one particular invader. For instance, this tweaked microbe might scout for — and attack — the bacterium responsible for cholera. Its infection causes life-threatening diarrhea.
Juan Borrero’s team at the University of Minnesota in Minneapolis recently tried something similar. They engineered harmless lactic acid bacteria to sense and destroy a disease-causing gut bacterium called Enterococcus faecalis (EN-tur-oh-KOK-us FEE-kah-lis).
To test if the newly modified bacteria could do their job, the researchers grew two batches of E. faecalis. They added their modified lactic acid bugs to one batch. And the bad bacteria in this batch only grew 25 to 50 percent as much as did those in the untreated batch. Borrero’s group shared its success June 4 in the journal ACS Synthetic Biology.
Help in the fight against drug-resistant germs also might come straight from the natural environment. Scientists in Canada have just announced their discovery of a potential substance that can turn off antibiotic resistance.
Gerard Wright of McMaster University in Hamilton, Ontario, and his co-workers isolated the substance from a soil fungus in eastern Canada. The fungus makes a chemical that disarms a gene in certain drug-resistant bacteria. That gene had allowed the bacteria to survive certain antibiotics. The researchers described their achievement June 25 in the journal Nature.
Such findings suggest that in some cases, resistance can be switched off. And that might restore former wonder drugs into miracle cures.
What have we learned?
Around 1930, Alexander Fleming was working at St. Mary’s Hospital in London, England. There he discovered a mold — Penicillium — that killed certain disease-causing bacteria. From that mold, Fleming created the antibiotic penicillin. A few years later, a second major class of antibiotics came along, known as sulfa drugs. Together, these early antibiotics launched a new era in medicine.
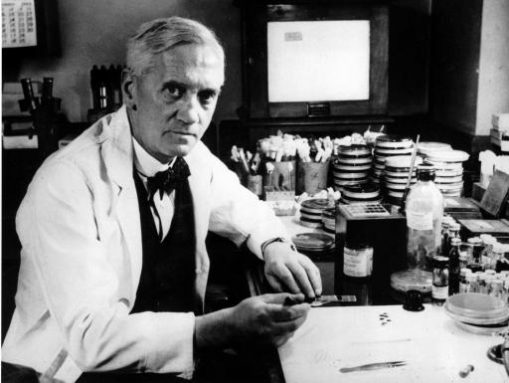
But the miracle is fading. Antibiotic resistance is a serious problem. The high-tech approaches now being used to fight this resistance are costly. Some scientists believe they would not have been needed, at least not for a long while, if people had used antibiotics more sparingly.
And doctors fear — truly fear — that, despite researchers’ efforts, a time may be quickly approaching when common diseases will again turn deadly, with no hope of a cure. That’s why they now argue that it’s the job of everyone — from scientist and doctor to patient, farmer and grocery shopper — to see that antibiotics are used when necessary, but only when necessary.
Power Words
admitted (in medicine) A term that refers to patients who are checked into a hospital — for a day or more — to be watched over or to receive treatment.
antibiotic A germ-killing substance prescribed as a medicine (or sometimes as a feed additive to promote the growth of livestock). It does not work against viruses.
bacterium(plural bacteria) A single-celled organism forming one of the three domains of life. These dwell nearly everywhere on Earth, from the bottom of the sea to inside animals.
bioengineer A researcher who applies technology for the beneficial manipulation of living things. Bioengineers use the principles of biology and the techniques of engineering to design organisms or products that can mimic, replace or augment the chemical or physical processes present in existing organisms. This field includes researchers who genetically modify organisms, including microbes. It also includes researchers who design medical devices such as artificial hearts and artificial limbs.
cholera A bacterial disease that infects the small intestine, causing sever diarrhea, vomiting and dehydration. It is spread by germs from feces that contaminate water or food.
DNA (short for deoxyribonucleic acid) A long, spiral-shaped molecule inside most living cells that carries genetic instructions. In all living things, from plants and animals to microbes, these instructions tell cells which molecules to make.
epidemiologist Like health detectives, these researchers figure out what causes a particular illness and how to limit its spread.
fungus (plural: fungi) Any of a group of unicellular or multicellular, spore-producing organisms that feed on organic matter, both living and decaying. Molds, yeast and mushrooms are all types of fungi.
gene A segment of DNA that codes, or holds instructions, for producing a protein. Offspring inherit genes from their parents. Genes influence how an organism looks and behaves.
germ Any one-celled microorganism, such as a bacterium, fungal species or virus particle. Some germs cause disease. Others can promote the health of higher-order organisms, including birds and mammals. The health effects of most germs, however, remain unknown.
hospitalist A type of medical doctor that works solely in a hospital, taking care of the patients throughout the time they are admitted to that hospital.
immune system The collection of cells and their responses that help the body fight off infection.
infection A disease that can be transmitted between organisms.
influenza (or flu) A highly contagious viral infection of the respiratory passages causing fever and severe aching. It often occurs as an epidemic.
microbe Short formicroorganism. A living thing that is too small to see with the unaided eye, including bacteria, some fungi and many other organisms such as amoebas. Most consist of a single cell.
microbiology The study of microorganisms, principally bacteria, fungi and viruses. Scientists who study microbes and the infections they can cause or ways that they can interact with their environment are known as microbiologists.
mutation Some change that occurs to a gene in an organism’s DNA. Some mutations occur naturally. Others can be triggered by outside factors, such as pollution, radiation, medicines or something in the diet. A gene with this change is referred to as a mutant.
phage Short for bacteriophage.This is a type of virus that infects — and ultimately kills — bacteria, but not before reproducing and spreading.
penicillin The first antibiotic (although not the first one used on people), it’s a natural product that comes from a mold.
pneumonia A lung disease in which infection by a virus or bacterium causes inflammation and tissue damage. Sometimes the lungs fill with fluid or mucus. Symptoms include fever, chills, cough and trouble breathing.
proteins Compounds made from one or more long chains of amino acids. Proteins are an essential part of all living organisms. They form the basis of living cells, muscle and tissues; they also do the work inside of cells. The hemoglobin in blood and the antibodies that attempt to fight infections are among the better known, stand-alone proteins.Medicines frequently work by latching onto proteins.
resistance (as in drug resistance) The reduction in the effectiveness of a drug to cure a disease, usually a microbial infection. (as in disease resistance) The ability of an organism to fight off disease.
superbug A disease-causing germ that can withstand medicines.
tuberculosis Best known as TB. A bacterial disease that causes unusual growths in the lungs or other tissues. Untreated, it can kill. The infection usually spreads when a sick individual coughs (or talks, sings or sneezes), spewing germs into the air.
virus iny infectious particles consisting of RNA or DNA surrounded by protein. Viruses can reproduce only by injecting their genetic material into the cells of living creatures. Although scientists frequently refer to viruses as live or dead, in fact no virus is truly alive. It doesn’t eat like animals do, or make its own food the way plants do. It must hijack the cellular machinery of a living cell in order to survive.