Stem cells: The secret to change
Unusual, versatile cells hold the key to regrowing lost tissues
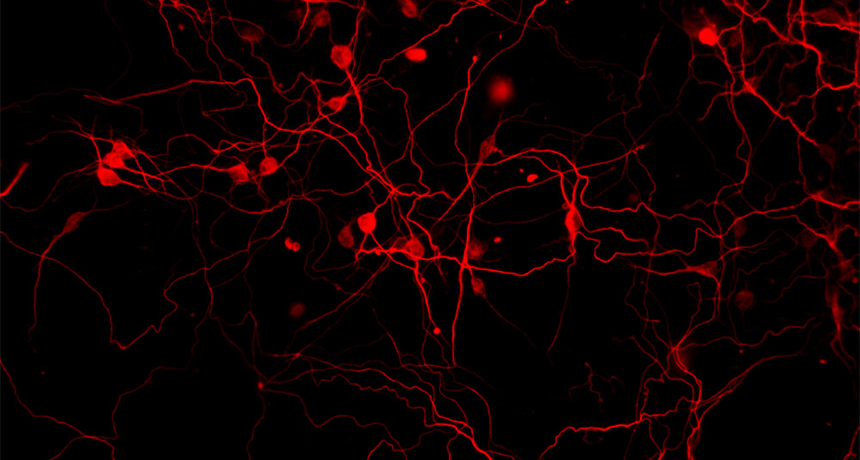
Neurons created from induced stem cells in Iqbal Ahmad’s lab glow red with fluorescent dye. The neuroscientist at the University of Nebraska Medical Center is researching whether the nerve cells could one day help restore sight to patients with glaucoma. Once injected in a patient, the nerve cells would work by inserting themselves between the retina and optic nerve, restoring signals to the brain.
Courtesy of Iqbal Ahmad
Inside your body, red blood cells are constantly on the move. They deliver oxygen to every tissue in every part of your body. These blood cells also cart away waste. So their work is crucial to your survival. But all that squeezing through tiny vessels is tough on red blood cells. That’s why they last only about four months.
Where do their replacements come from? Stem cells.
These are a very special family of cells. When most other cells divide, the daughter cells look and act exactly like their parents. For example, a skin cell can’t make anything but another skin cell. The same is true for cells in the intestine or liver.
Not stem cells. Stem cells can become many different types. That is how an embryo grows from a single fertilized egg into a fetus with trillions of specialized cells. They need to specialize to make up tissues that function very differently, including those in the brain, skin, muscle and other organs. Later in life, stem cells also can replace worn-out or damaged cells — including red blood cells.
The remarkable abilities of stem cells make them very exciting to scientists. One day, experts hope to use stem cells to repair or replace many different kinds of tissues, whether injured in accidents or damaged by diseases. Such stem cell therapy would allow the body to heal itself. Scientists have found a way to put specialized cells to work repairing damage, too. Together, these cell-based therapies might one day make permanent disabilities a thing of the past.
One unusual type of stem cell offers special promise for such therapeutic uses. For the recent development of this cell type, Shinya Yamanaka shared the 2012 Nobel Prize in medicine.
Meet the family
Blood stem cells live inside your bones, in what is called marrow. There, they divide over and over. Some of the new cells remain stem cells. Others form red blood cells. Still others morph into any of the five types of white blood cells that will fight infections. Although blood stem cells can become any one of these specialized blood cells, they cannot become muscle, nerve or other types of cells. They are too specialized to do that.
Another type of stem cell is more generalized. These can mature into any type of cell in the body. Such stem cells are called pluripotent (PLU ree PO tint). The word means having many possibilities. And it’s not hard to understand why these cells have captured the imaginations of many scientists.
Until recently, all pluripotent cells came from embryos. That’s why scientists called them embryonic stem cells. After an egg is fertilized, it divides in two. These two cells split again, to become four cells, and so on. In the first few days of this embryo’s development, each of its cells is identical to all the others. Yet each cell has the potential to develop into any specialized cell type.
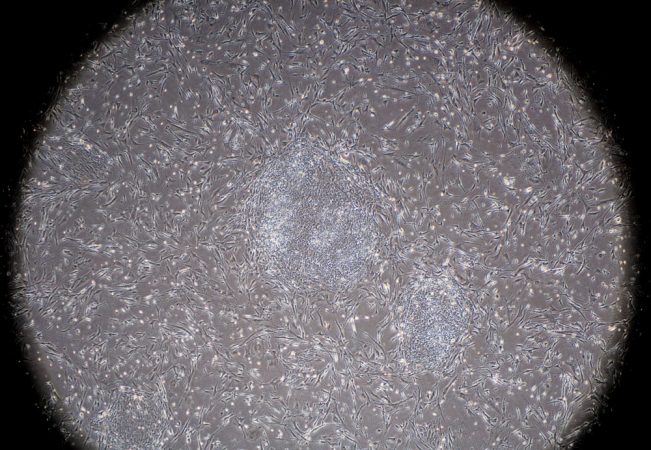
When the human embryo reaches three to five days old, its cells start to realize their potential. They specialize. Some will develop into muscle cells or bone cells. Others will form lung cells — or maybe the cells lining the stomach. Once cells specialize, their “many possibilities” suddenly become limited.
By birth, almost all of a baby’s cells will have specialized. Each cell type will have its own distinctive shape and function. For example, muscle cells will be long and able to contract, or shorten. Red blood cells will be small and plate-shaped, so they can slip through blood vessels with ease.
Hidden among all of these specialized cells are pockets of adult stem cells. (Yes, even newborns have “adult” stem cells.) Unlike embryonic stem cells, adult stem cells cannot transform into any and every cell type. However, adult stem cells can replace several different types of specialized cells as they wear out. One type of adult stem cell is found in your marrow, making new blood cells. More types are found in other tissues, including the brain, heart and gut.
Among naturally occurring stem cells, the embryonic type is the most useful. Adult stem cells just aren’t as flexible. The adult type also is relatively rare and can be difficult to separate from the tissues in which it is found. Although more versatile, embryonic stem cells are both difficult to obtain and controversial. That’s because harvesting them requires destroying an embryo.
Fortunately, recent discoveries in stem cell research now offer scientists a third — and potentially better — option.
The search for answers
In 2006, Shinya Yamanaka discovered that specialized cells — like those in skin — could be converted back into stem cells. Working at Kyoto University in Japan, this doctor and scientist induced — or persuaded — mature cells to become stem cells. He did this by inserting a specific set of genes into the cells. After several weeks, the cells behaved just like embryonic cells. His new type of stem cells are called induced pluripotent stem cells, or iP stem cells (and sometimes iPS cells).
Yamanaka’s discovery represented a huge leap forward. The iP stem cells offer several advantages over both embryonic and adult stem cells. First, iP stem cells are able to become any cell type, just as embryonic stem cells can. Second, they can be made from any starting cell type. That means they are easy to obtain. Third, in the future, doctors would be able to treat patients with stem cells created from their own tissues. Such cells would perfectly match the others, genetically. That means the patient’s immune system (including all of its white blood cells) would not attack the introduced cells. (The body often mounts a life-threatening attack against transplanted organs that come from other people because they don’t offer such a perfect match. To the body, they seem foreign and a potentially dangerous “invader.”)
Scientists the world over learned of the technique developed by Yamanaka (who now works at the Gladstone Institutes — which is affiliated with the University of California, San Francisco). Many of these researchers adopted Yamanaka’s procedure to create their own induced pluripotent stem cells. For the first time, researchers had a tool that could allow them to make stem cells from people with rare genetic diseases. This helps scientists learn what makes certain cell types die. Experts can also expose small batches of these diseased cells to different medicines. This allows them to test literally thousands of drugs to find out which works best.
And in the future, many experts hope induced stem cells will be used to replace adult stem cells and the cells of tissues that are damaged or dying.
Therapies take patients — and patience
Among those experts is Anne Cherry, a graduate student at Harvard University. Cherry is using induced stem cells to learn more about a very rare genetic disease called Pearson syndrome. A syndrome is a group of symptoms that occur together. One symptom of Pearson syndrome is that stem cells in bone marrow cannot make normal red blood cells. This condition typically leads to an early death.
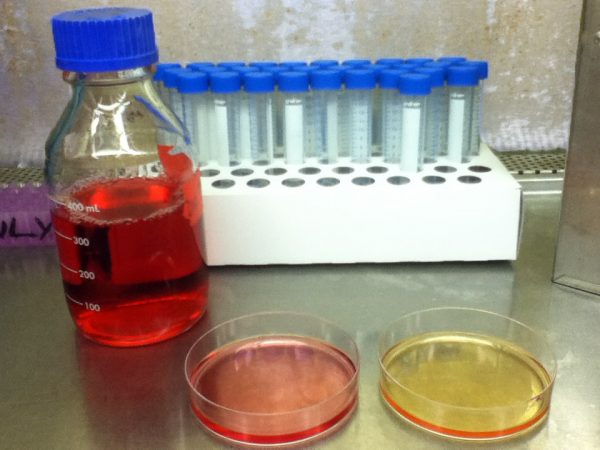
Cherry has begun to study why these stem cells fail.
She started by taking skin cells from a girl with the disease. She placed the cells in a test tube and added genes to turn them into stem cells. Over several weeks, the cells began to make proteins for which the inserted genes had provided instructions. Proteins do most of the work inside cells. These proteins turned off the genes that made the cells act like skin cells. Before long, the proteins turned on the genes to make these cells behave like embryonic stem cells.
After about three months, Cherry had a big batch of the new induced stem cells. Those cells now live in Petri dishes in her lab, where they are kept at body temperature (37° Celsius, or 98.6° Fahrenheit). The scientist is now trying to coax the induced stem cells into becoming blood cells. After that, Cherry wants to find out how Pearson syndrome kills them.
Meanwhile, the patient who donated the skin cells remains unable to make blood cells on her own. So doctors must give her regular transfusions of blood from a donor. Though life-saving, transfusions come with risks, particularly for someone with a serious disease.
Cherry hopes to one day turn the girl’s induced stem cells into healthy new blood stem cells — and then return them to the girl’s body. Doing so could eliminate the need for further transfusions. And since the cells would be the girl’s own, there would be no risk of her immune system reacting to them as though they were foreign.
Sight for sore eyes
At University of Nebraska Medical Center in Omaha, Iqbal Ahmad is working on using stem cells to restore sight to the blind. A neuroscientist — someone who studies the brain and nervous system — Ahmad has been focusing on people who lost sight when nerve cells in the eye’s retina died from a disease called glaucoma (glaw KOH muh).
Located inside the back of the eye, the retina converts incoming light into electrical signals that are then sent to the brain. Ahmad is studying how to replace dead retina cells with new ones formed from induced pluripotent stem cells.
The neuroscientist starts by removing adult stem cells from the cornea, or the clear tissue that covers the front of the eye. These stem cells normally replace cells lost through the wear and tear of blinking. They cannot become nerve cells — at least not on their own. Ahmad, however, can transform these cells into iP stem cells. Then, with prodding, he turns them into nerve cells.
To make the transformation, Ahmad places the cornea cells on one side of a Petri dish. He then places embryonic stem cells on the other side. A meshlike membrane separates the two types of cells so they can’t mix. But even though they can’t touch, they do communicate.
Cells constantly send out chemical signals to which other cells respond. When the embryonic stem cells “speak,” the eye cells “listen.” Their chemical messages persuade the eye cells to turn off the genes that tell them to be cornea cells. Over time, the eye cells become stem cells that can give rise to different types of cells, including nerve cells.
When Ahmad’s team implanted the nerve cells into the eyes of laboratory mice and rats, they migrated to the retina. There, they began replacing the nerve cells that had died from glaucoma. One day, the same procedure may restore vision to people who have lost their sight.
Another approach
In using a body’s own cells to repair injury or to treat disease, stem cells aren’t always the answer. Although stem cells offer tremendous advances in regenerating lost tissue, some medical treatments may work better without them. That’s thanks to the chemical communication going on between all cells all of the time. In some situations, highly specialized cells can act as a conductor, directing other cells to change their tune.
In 2008, while working at the University of Cambridge in England, veterinary neurologist Nick Jeffery began a project that used cells taken from the back of the nose. But Jeffery and his team were not out to create stem cells. Instead, the scientists used those nasal cells to repair damaged connections in the spinal cord.
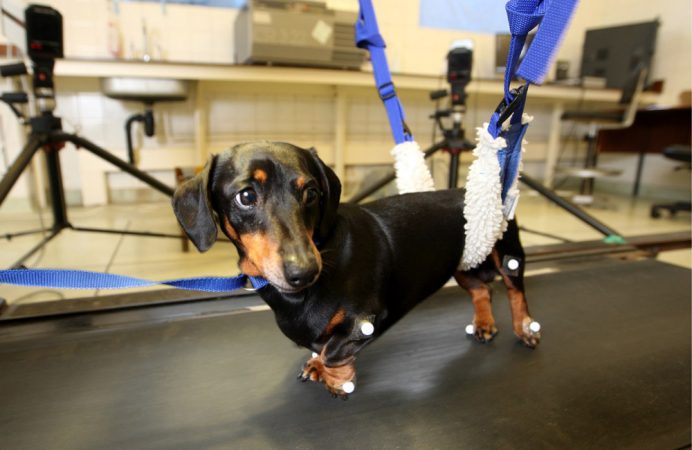
The spinal cord is basically a rope of nerve cells that ferry signals to and from the brain and other parts of the body. Injuring the spinal cord can lead to paralysis, or the loss of sensation and the inability to move muscles.
Like Ahmad, some researchers are using stem cells to replace damaged nerve cells. But Jeffery, now at Iowa State University in Ames, doesn’t think such techniques are always necessary to aid recovery from spinal injuries. “Stem cell transplantation,” points out Jeffery’s colleague, neuroscientist Robin Franklin, “is to replace a missing cell type.” In a spinal injury, the nerve cells aren’t missing. They’re just cut off.
Nerve cells contain long, wirelike projections — called axons — that relay signals to the next cell. When the spine is injured, these axons can become severed, or cut. Damaging an axon is like snipping a wire — the signal stops flowing. So the Cambridge scientists set out to see if they could restore those signals.
Jeffery and his fellow scientists work with dogs that have experienced spinal injuries. Such problems are common in some breeds, including dachshunds. The team first surgically removed cells from the dogs’ sinuses — or the hollow spaces in the skull behind the nose. These are not stem cells. These particular cells instead encourage nerve cells in the nose to grow new axons. These cells help the pooches maintain their healthy sense of smell.
The scientists grew these sinus cells in the lab until they had reproduced to large numbers. Then the researchers injected the cells into the spinal cords of two out of every three doggy patients. Each treated dog received an injection of its own cells. The other dogs got an injection of only the liquid broth used to feed the growing cells.
Over several months, the dogs’ owners repeatedly brought their pets back to the lab for testing on a treadmill. This allowed the scientists to evaluate how well the animals coordinated their front and hind feet while walking. Dogs that had received the nasal cells steadily improved over time. Dogs that received only the liquid did not.
This treatment did not result in a perfect cure. Nerve cells did reconnect several portions of the spinal cord. But nerve cells that once linked to the brain remained disconnected. Still, these dog data indicate that nasal cells can aid in recovering from a spinal cord injury.
Such new developments in cellular research suggest that even more remarkable medical advancements may be just a few years away. Yamanaka, Cherry, Ahmad, Jeffery, Franklin and many other scientists are steadily unlocking secrets to cellular change. And while you can’t teach an old dog new tricks, scientists are finding out that the same just isn’t true of cells anymore.
Power Words
cornea The clear covering over the front of the eye.
embryo A vertebrate, or animal with a backbone, in its early stages of development.
gene A section of DNA that carries the genetic instructions for making a protein. Proteins do most of the work in cells.
glaucoma An eye disease that damages nerve cells carrying signals to the brain.
immune cell White blood cell that helps protect the body against germs.
molecule A collection of atoms.
neuron (or nerve cell) The basic working unit of the nervous system. These cells relay nerve signals.
neuroscientist A researcher who studies neurons and the nervous system.
paralysis Loss of feeling in some part of the body and an inability to move that part.
retina The light-sensitive lining at the back of the eye. It converts light into electrical impulses that relay information to the brain.
sinus An opening in the bone of the skull connected to the nostrils.
spinal cord The ropelike collection of neurons that connect the brain with nerves throughout the body.
tissue A large collection of related, similar cells that together work as a unit to perform a particular function in living organisms. Different organs of the human body, for instance, often are made from many different types of tissues. And brain tissue will be very different from bone or heart tissue.
transfusion The process of transferring blood into one person that had been collected from another.
Word Find (click here to enlarge puzzle for printing)